Phytosterol supplementation enhances the growth performance, feed utilization, antioxidant status and glucose metabolism of juvenile largemouth bass (Micropterus salmoides) fed a high-starch diet
- Zhejiang Fisheries Technical Extension Center, Hangzhou, China
Introduction: Previous studies found that phytosterols could influence growth performance, feed utilization and lipid metabolism as well as improve the antioxidant capacity of animals.
Methods: To investigate the effects of dietary phytosterol supplementation on juvenile largemouth bass (Micropterus salmoides) fed a high-starch diet, a 56- day feedingtrial was conducted with four dietary feeds for juvenile largemouth bass: extruded floating feed isonitrogenous and isoenergetic diets were formulated to contain 10% and 15% α-starch; on the basis of a 15% α-starch diet, two other diets were formulated with supplementation of 0.1% and 0.5% phytosterol, respectively. After the feeding trials, the survival rate, weight gain and specific growth rate, feed conversion ratio, intraperitoneal fat ratio, feed intake, protein efficiency ratio and activities of three digestive enzymes, as well as the concentrations of nine plasma biochemical indices, hepatic enzyme activities and glycogen contents, were measured and calculated, and the data were statistically analyzed.
Results: The results of the present study showed that the survival rate, weight gain and specificgrowthrates were significantly greater in plants fed high-starch diets supplemented with phytosterols. As the supplemental phytosterol concentration increased, the feed conversion ratio and intraperitoneal fat ratio significantly decreased; the protease and lipase levels in the pyloric zone markedly increased; the plasma cholesterol, triglyceride, glucose, malondialdehyde, aspartate transaminase and alanin transaminase levels significantly decreased; the glucokinase and pyruvate kinase levelsmarkedly increased; and the hepatic glycogen content significantly decreased.
Discussion: In summary, dietary phytosterol supplementation promoted the growth performance, feed utilization and antioxidant status of juvenile largemouth bass fed a highstarch diet; enhanced glucose utilization and metabolism; and alleviated the negative stimulation of glycemia stress in M. salmoide fed a high-starch diet.
1 Introduction
As the most economical energy source in aquatic feeds, starch can effectively reduce dietary protein and lipids (Zhou et al., 2022). Moreover, starch plays a prominent role in feed processing, especially for extruded floating pellets, which are typically used as stabilizers and swelling agents (Hemre et al., 2002). Hence, starch is widely used in various practical diet ingredients. However, the ability of fish to utilize starch is limited and related to the fish species. Compared with omnivorous and herbivorous fish, carnivorous fish have a lower ability to make use of dietary carbohydrates. Excessive dietary carbohydrates generally cause persistent glycogen deposition (Wu et al., 2016; Lin et al., 2018; Ma et al., 2019), induce an immune response (Hu et al., 2017; Cheng et al., 2020; Borin-Crivellenti et al., 2021) and further impair the growth and health status of cultured carnivorous fish. Therefore, studies on how to alleviate hepatic glycogen deposition caused by high dietary carbohydrates, which may contribute to coping with the nutritional–technological conundrum in manufacturing aquafeeds of carnivorous fish, should receive much attention.
Largemouth bass, Micropterus salmoides, has been widely cultured in China and has become one of most commercially important carnivorous species for freshwater culture (Li et al., 2021; Liang et al., 2022), and its production increased rapidly from 175 thousand tons in 2009 to approximately 702,093 tons in 2021 (Hou et al., 2023). In recent years, M. salmoides fed a commercial diet instead of fresh/thawed raw fish has been widely accepted by farmers in Zhejiang Province, for which the output of M. salmoides, which exceeds 95% of M. salmoides aquafarms using extruded floating feed, is ranked 2nd in China. However, M. salmoides shows poor utilization of dietary carbohydrates (Ma et al., 2019; Chen et al., 2023), and a starch-rich diet may lead to abnormal hepatic glycogen reservation and lipid metabolism and further impair the health status and growth performance of the fish.
Phytosterols (PSs), which include plant sterols and stanols, are steroid compounds similar to cholesterol that occur in plants and are often enriched in seeds and seed oils. Many investigators have demonstrated the cholesterol-lowering effects of phytosterols in chickens (Zhao et al., 2019), broilers (Naji et al., 2014; Ding et al., 2021) and rats (Marineli et al., 2012). In addition, it has been illustrated in previous reports that phytosterols in the diet could accelerate the growth rate and/or improve the meat quality of broilers and ducks (Wu et al., 2012; Naji et al., 2014). Recently, phytosterols have received increasing attention in the field of antioxidants (Trautwein and Demonty, 2007; Xie et al., 2015). However, little is known about the effects of dietary phytosterols on fish. The use of vegetable oils in feeds for farmed Atlantic salmon (Salmo salar) has increased in recent years (Liland et al., 2013). Phytosterols may also affect fish health through potential effects on cholesterol metabolism and liver lipid levels (Couto et al., 2014; He et al., 2022; Reyes-Becerril et al., 2022).
To date, limited reports are available regarding the effects of dietary phytosterol inclusion on M. salmoides. Therefore, the objective of the present study was to evaluate the effects of phytosterol supplementation in a high-starch diet on the growth performance and glucose and lipid metabolism response of juvenile M. salmoides. It is assumed that the findings of the present study will provide a basis for alleviating hepatic glycogen deposition and improving antioxidant status, which might contribute to more efficient formulation of economical diets for M. salmoides.
2 Materials and methods
2.1 Preparation of diets
Two extruded floating-feed isonitrogenous (46% crude protein) and isoenergetic (13 kJ/g) diets were formulated to contain 10% (w/w, LS) and 15% (w/w, HS) α-starch, and the other two diets were formulated supplemented with 0.1% (w/w) and 0.5% (w/w) phytosterol, respectively, on the basis of the HS diet (Table 1). The extruded feed was produced by Hangzhou Haihuang Group Co., Ltd. (Hangzhou, Zhejiang, China). All ingredients were ground through a 320 μm mesh before final mixing through a commercial food mixer. Feeds were extruded into 2 mm diameter pellets under the following extrusion conditions: the barrel temperature was adjusted to 100 and 105°C, the moisture content was 28% and 35%, and the screw speed was 40–45 rpm using a Twin-screwed extruder (HR118, HENGRUN MACHINE, Zhanjiang, China). The extruded fish feed was dried at 40°C for spraying oil and ground into powder to pass through a 50 mm mesh sieve. All diets were stored at room temperature (25–30°C) in the summer and kept out of the sun and rain. The diet formulation and proximate composition are shown in Table 1.
2.2 Experimental treatments
A 56-day feeding trial was conducted at the indoor cyclic water system of the Hangzhou Xiba field station, which belongs to the Zhejiang Fisheries Technical Extension Center (Hangzhou, Zhejiang, China). M. salmoides juveniles were obtained from the commercial fish hatchery of Huzhou (Zhejiang, China) and acclimatized to laboratory conditions with commercial feed (Hangzhou Haihuang Group Co., Ltd., China) for 14 days. At the start of the trial, the fish were starved for 24 h and weighed after being anesthetized with 100 mg/L MS-222 (tricaine methane sulfonate, Sigma, St. Louis, MO, USA). The experimental fish (mean initial weight: 53.78 ± 1.13 g) were randomly allocated into 9 cylindrical plastic tanks (capacity: 500 L) for the growth trial (20 fish per tank). Each dietary treatment group was randomly assigned to triplicate tanks. The fish were fed to apparent satiation by hand two times (08:00 and 17:00) daily for 8 weeks. No feed residue was left in the tank after feeding to ensure that the fish could eat the diet. Each tank was supplied with recycled freshwater at a flow rate of 2 L min−1. During the trial, the water temperature ranged from 27.5 to 29.5°C, the ammonia-N concentration was less than 0.20 mg/L, the dissolved oxygen concentration was greater than 6.0 mg/L, and the pH ranged from 7.1~7.3. The photo period was 12 L:12 D, with a light period from 07:00 to 19:00.
2.3 Sample collection
At the end of the trial, the fish were fasted for 24 h before harvest. All experimental fish were anesthetized with MS-222 at 0.1 g kg-1 and then weighed and counted individually. Blood samples from six fish per tank were collected via the caudal vein using a 1-mL syringe with a 27-gauge needle. Blood samples were immediately centrifuged (4500 rpm, 10 min) at 4°C (Eppendorf centrifuge 5430 R, Hamburg, Germany), and the plasma was separated and stored at -20°C for further analysis. The bloodless fish were then dissected to obtain the viscera and liver for calculating the parameters of the conditions. The dorsal muscle, liver and intestine of the fish were rapidly frozen in liquid nitrogen and stored at -80°C.
2.4 Biochemical analysis
The experimental diets and tissues were analyzed in triplicate for proximate composition. The moisture, ash, crude protein and crude lipid contents were determined following methods of the Association of Official Analytical Chemists. The moisture concentration was determined by drying minced samples for 6 h in a forced-air oven maintained at 105°C. The ash content was analyzed by incinerating the samples at 600°C for 24 h in a muffle furnace. Crude protein was estimated as Kjeldahl nitrogen using a factor of 6.25, and crude lipid content was determined by Soxhlet extraction with petroleum ether for 6 h.
The plasma biochemical indices, including total protein (TP), total cholesterol (TCH), triglyceride (TG) and glucose (GLU) contents; immune and antioxidant indices, including superoxide dismutase (SOD), catalase (CAT), alanine aminotransferase (ALT) and aspartate transaminase (AST) activities; and malondialdehyde (MDA) content in plasma, were assayed by commercial kits. The activities of key carbohydrate-metabolizing enzymes, such as glucokinase (GK), hexokinase (HK), pyruvate kinase (PK), phosphoglucokinase (PEK) and phosphoenolpyruvate carboxykinase (PEPCK), in the liver, as well as digestive enzymes (pepsin, lipase and amylase) in the stomach, pyloric region and intestine, and hepatic and muscle glycogen of the experimental fishes, were measured within 3 days. The above trials all used commercial diagnostic reagent kits purchased from Nanjing Jiancheng Bioengineering Institute (Nanjing, Jiangsu Province, P. R. China) according to the manufacturer’s instructions. All assays were performed in triplicate.
2.5 Statistical analysis
All the data are presented as the mean ± SE of three replicate groups and were analyzed by using SPSS software version 18.0 for Windows. Differences between groups were evaluated by one-way ANOVA followed by Tukey’s multiple comparison test. Statistical significance was set at P < 0.05.
The following variables were calculated:
Weight gain (WG) (%)=100%×(FBW−IBW)/IBW
Specific growth rate (SGR) (%/day)=100%×(ln FBW−ln IBW)/t
Survival (%)=100%×Nt/N0
Feed intake (FI) (g/day)=dry feed consumed (g)/t
Feed conversion ratio (FCR)=dry feed intake (g)/(FBW−IBW)
Hepatosomatic index (HSI) (%)=100%×(FLW/FBW)
Viscera index (VSI) (%)=100%×(FVW/FBW)
Condition factor (CF) (g/cm3)=100%×FBW/L3
Protein efficiency ratio (PER) (%)=(FBW−IBW)/dry protein intake (g)
Intraperitoneal fat ratio (IPFR) (%)=100%×intraperitoneal fat weight (g)/FBW
IBW (g): initial body weight; FBW (g): final body weight; FLW (g): final liver weight; FVW (g): final viscera weight.
3 Results
3.1 Growth performance and feed utilization
The growth performance of the experimental M. salmoides is shown in Table 2. Survival was significantly lower in the fish fed the HS diet (P<0.05); however, when the fish were fed the diets supplemented with phytosterols, no significant difference in survival was found compared with those fed the control diet. The fish in the HS group exhibited the lowest weight gain (WG) and specific growth rate (SGR) among all the treatments (P<0.05). The WG and SGR of M. salmoides in the control group and phytosterol-treated groups were significantly greater than those in the HS group (P<0.05), and no significant differences were detected between the fish in the 0.1% and 0.5% PS diets. Compared with those in the control group, the fish in the HS group had significantly lower feed intake (FI) and protein efficiency ratio (PER) and the highest feed conversion ratio (FCR) (P<0.05), while no significant differences were found among the fish fed the diets supplemented with 0.1% or 0.5% PS. In addition, no significant difference was found in the FCR or PER between the HS+0.1% PS group and the control group. Morphometric parameters were mostly not related to dietary treatments, with the exception of the IPFR of fish fed the HS diet, which was much greater than the values obtained in the other groups (P<0.05) (Table 2).
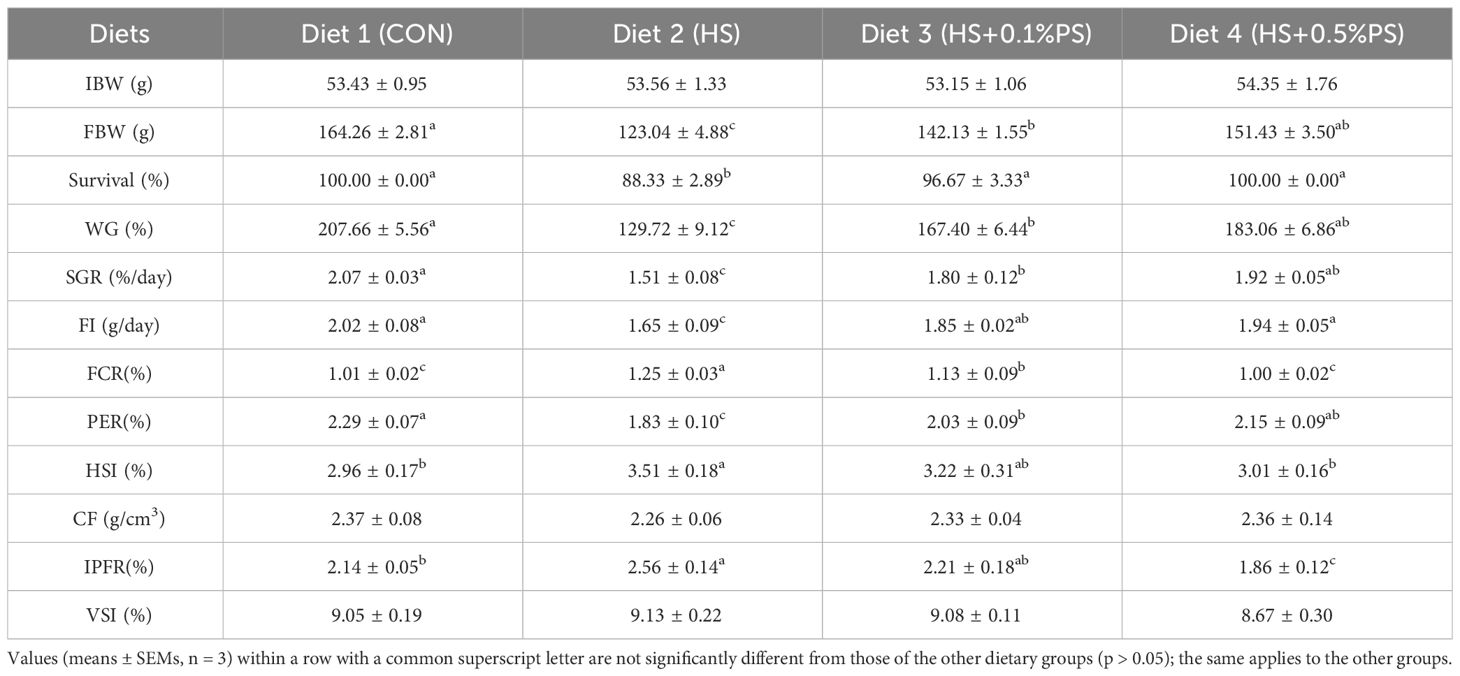
Table 2 Growth performance and feed utilization of juvenile M. salmoides fed experimental diets for 8 weeks.
3.2 Composition of the dorsal muscle
No significant difference was observed in the moisture or crude ash content in the dorsal muscle among the dietary treatments (Table 3). However, the muscle crude protein content in the experimental fish in the HS and HS+0.1% PS groups was lower than that in the other groups (P<0.05). The highest and lowest lipid contents were found in fish fed the HS and HS+0.5% PS diets (P<0.05), respectively (Table 3).

Table 3 Effects of experimental diets on the dorsal muscle composition of juvenile M. salmoides (%).
3.3 Activities of digestive enzymes
The activities of digestive enzymes in the stomach, pylorus and intestine are shown in Table 4. The lowest and highest activities of pepsin in the stomach were found in M. salmoides in the HS and control groups (P<0.05), respectively. However, dietary phytosterol supplementation increased the pepsin activity of the fish, and there was no significant difference between the fish fed the HS+0.5% PS diet and those fed the control diet. The lipase and amylase activities in the stomach remained stable, and no significant differences existed among the experimental groups. In the pyloric group, relatively greater lipase and pepsin activities were detected in the fish in the phytosterol supplementation groups (P<0.05), while the amylase activity was not affected by the dietary treatments. Protease activity in the intestine was greater in fish fed the LS diet than in those fed the other diets (P<0.05), but no significant difference was found in fish fed the high-starch diet. The lipase and amylase activities were not significantly different among the dietary treatments (Table 4).
3.4 Concentrations of plasma biochemical indices
The effects of dietary treatments on the plasma biochemical indices of M. salmoides are presented in Table 5. When the fish were fed the HS diet, the plasma TG and GLU contents were significantly greater than those in the fish fed the control diet (P<0.05). Moreover, as the dietary PS concentration increased from 0.1% to 0.5%, the two parameters gradually decreased. The highest and lowest contents of TCH were detected in fish fed the HS and HS+0.5%PS diets (P<0.05), respectively. In terms of antioxidant capacity, the fish fed the HS diet had a greater content of MDA (P<0.05), and no significant difference was found among the other treatments (Table 5). ALS and AST activities in fish fed the HS diet were significantly greater than those in the control diet and HS+0.5%PS diet (P<0.05); however, no significant difference was detected between the HS and HS+0.1%PS groups. In addition, the contents of TP, SOD and CAT were not affected by the dietary treatments.
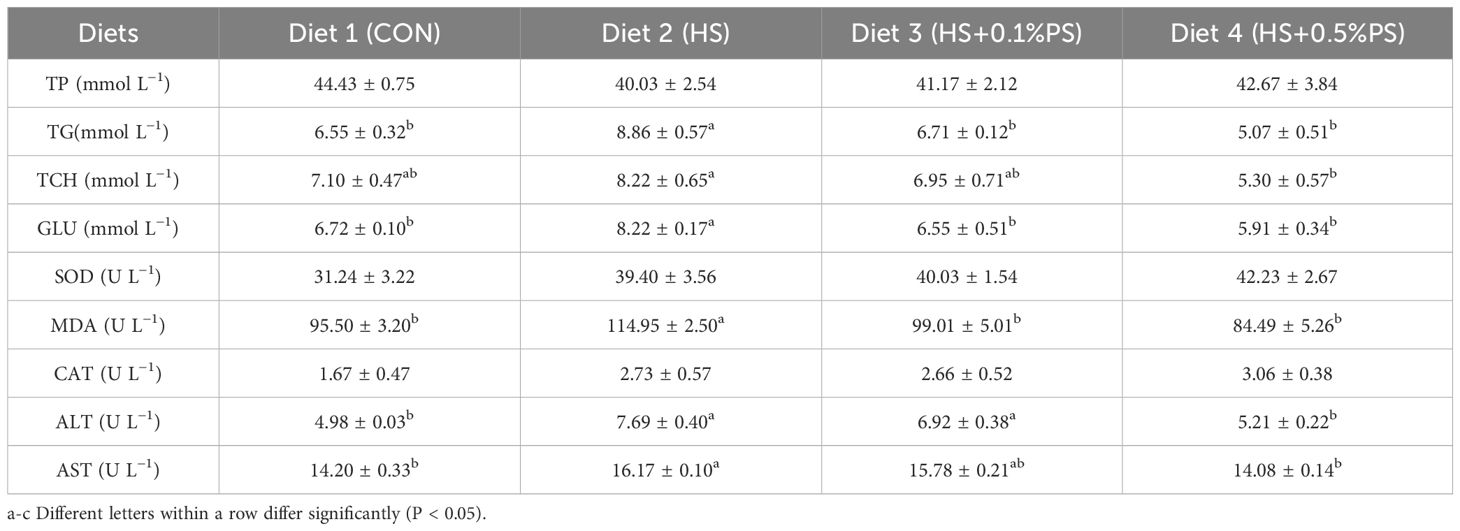
Table 5 Effects of experimental diets on the concentrations of biochemical indices in the plasma of juvenile M. salmoides.
3.5 Activities of hepatic carbohydrate metabolic enzymes
The activities of carbohydrate metabolism-related enzymes in the liver of M. salmoides responded differently to the experimental diets (Table 6). The levels of key gluconeogenesis enzymes, including GK and PK, showed a similar increasing trend as the PS level increased in the HS diet group (P<0.05), while no significant difference was found in the HK activity compared with that in the control diet group. The activities of the hepatic gluconeogenesis enzymes PEK and PEPCK were irregular and not related to the dietary treatments.
3.6 Glycogen contents in the dorsal muscle and liver
The glycogen content in the dorsal muscle was relatively low, and there were no significant differences among the dietary treatments (Table 7). In contrast, the hepatic glycogen content was significantly influenced by dietary treatment. The highest and lowest glycogen contents in the liver were observed in the HS group and the control group, respectively (P<0.05). With the increase in dietary phytosterols from 0.1% to 0.5% in the high-starch diet group, the hepatic glycogen content tended to decrease, and the value was significantly greater than that in the control diet group (P<0.05).

Table 7 Muscle and hepatic glycogen content of juvenile M. salmoides fed diets with experimental diets for 8 weeks.
4 Discussion
Previous studies on partridge shank chickens (Zhao et al., 2019), broilers (Naji et al., 2014; Ding et al., 2021) and rats (Marineli et al., 2012) have indicated that phytosterols can influence growth performance, feed utilization, and lipid metabolism and improve the antioxidant capacity of animals and can also enhance immune responses and antimicrobial activity but exert few effects on growth performance in some fish species (Couto et al., 2014; He et al., 2022; Reyes-Becerril et al., 2022). In addition, phytosterol can alleviate the damage caused by a high-lipid diet (He et al., 2022). The limited starch utilization capacity of M. salmoides has been demonstrated in a number of previous studies, and body weight gain, feed efficiency, and hepatic or intestinal health status could be negatively influenced by excessive dietary carbohydrates (Lin et al., 2018; Li et al., 2019; Ma et al., 2019; Zhang et al., 2020; Huang et al., 2021). To alleviate the negative effects induced by a high-carbohydrate diet, researchers have identified several feed additives, such as bile acid (Yu et al., 2019), resveratrol (Liu et al., 2021), berberine (Xia et al., 2022) and sodium diacetate (Fang et al., 2024). By exploring the effects of phytosterols in M. salmoides diets with a high starch content for the first time, our study revealed that phytosterols could effectively decrease the negative impact on feed utilization efficiency and plasma biochemical indices of M. salmoides induced by a high-starch diet and could also promote weight gain and specific growth ratios of M. salmoides, which is different from reports on large yellow croaker (Larimichthys crocea), gilthead sea bream (Sparus aurata L.) and European sea bream (Dicentrarchus labrax). The difference might be attributed to differences in animal species, size, starch level and dose of phytosterols. In addition, our results revealed that as the dietary phytosterol concentration increased from 0.1% to 0.5%, the FCR and IPFR significantly decreased, which indicated that supplemental phytosterols could promote feed utilization by M. salmoides and that the phytosterol dosage appeared to be significantly associated with these effects. Digestive enzymes are important compounds that mediate nutritional processes, and their activities represent the ability of the organism to digest and absorb nutrients (Lundstedt et al., 2004). The activities of protease and lipase in pyloric plants tended to increase markedly with increasing dietary phytosterol levels, which may have contributed to the recovery of feed utilization efficiency from high starch stress. However, the amylase activities were relatively low and not affected by the dietary treatments. This result was in accordance with previous reports (Li et al., 2020).
The plasma biochemical index is an important indicator for assessing fish health and nutritional status and is involved in many metabolic processes (Fırat et al., 2011). In our study, except for total protein (TP), superoxide dismutase (SOD) and catalase (CAT), the concentrations of six plasma biochemical indices significantly changed after diet treatment. Phytosterols have been thoroughly proven to lower plasma cholesterol in mammals (Laraki et al., 1993; Matvienko et al., 2002; Doornbos et al., 2005; Couto et al., 2015). Our study revealed that phytosterols play a role in lowering the plasma cholesterol and triglyceride levels in M. salmoides fed a high-starch diet, which indicated that phytosterols promoted lipid metabolism, consistent with findings in juvenile large yellow croaker (He et al., 2022), Atlantic salmon (Salmo salar L.) (Liland et al., 2013) and bluntnose black bream (Megalobrama amblycephala) (Zhang et al., 2012). Any change in the physiological status of the fish, from pollution to nutritional stress, can cause changes in liver enzymes. The fish liver is a key organ of numerous vital metabolic reactions related to carbohydrates, lipids, and proteins. AST and ALT play important roles in protein and amino acid metabolism and may be released into the plasma following tissue damage and dysfunction, and they are regarded as indicators of hepatic injury in aquaculture animals (Wang et al., 2020; Zare et al., 2023). Our results revealed that AST and ALT both significantly increased in the high-starch diet group but tended to decrease with increasing phytosterol concentration. With an additive dose of 0.5%, the concentrations of the indices could revert to normal dietary conditions, indicating the potential protection of phytosterols against negative stimulation of the fish body induced by high starch stress. Similar results were found in trials of bile acids (Guo et al., 2020).
Antioxidative systems are one of the main physiological mechanisms for protecting the health of aquatic animals (Castex et al., 2009). Antioxidation eliminates active middle products, especially oxyradicals, to protect cells and molecules from the oxidative damage caused by the attack of radicals. As the first line of defense against oxidative stress (Ighodaro and Akinloye, 2018), SOD and CAT are important antioxidative enzymes that work together to catalyze free radicals. MDA is a generally accepted and widely used biomarker of oxidative stress, notably lipid peroxidation (Tsikas, 2017), and SOD and MDA are often used as effective indicators to objectively reflect the antioxidant status of animals (Shadrack et al., 2022). In our study, the concentrations of SOD and CAT increased with increasing phytosterol addition. Moreover, plasma MDA significantly increased in the high-starch diet group but tended to decrease with increasing phytosterol treatment, which revealed the beneficial effect of phytosterol supplementation on the antioxidant status of M. salmoides. Similar results were found in chickens (Zhao et al., 2019) and large yellow croaker (He et al., 2022). Taken together, the increased antioxidant enzyme activity paralleled the reduced level of MDA resulting from phytosterol supplementation, indicating that dietary phytosterols could improve the antioxidant status of M. salmoides.
Our study revealed that phytosterol decreased plasma glucose in accordance with findings for bile acids and berberine (Guo et al., 2020; Xia et al., 2022), which indicated the potential acceleration function of phytosterol in glucose metabolism. Moreover, the levels of five glucose metabolism-related enzymes in the liver of M. salmoides were affected by the dietary treatments. Glycolysis is an important route of glucose catabolism, and GK, PK and HK are enzymes that play key roles in the regulation of the glycolysis pathway (Enes et al., 2010). Similarly to those in a previous study, the activities of GK and PK increased significantly in plants fed a high-carbohydrate diet, as excess glucose activated PK to produce pyruvic acid (Tan et al., 2010). The low activity of HK in fish fed high starch might harm glucose utilization and induce glucose intolerance in largemouth bass. In our study, the activities of hepatic HK did not significantly increase in the groups fed high dietary starch after 56 days of feeding or in the groups supplemented with phytosterol, which might be attributed to the feedback of excess Glu-6-P produced by continuous glycemia inhibiting activity. This was in agreement with the results reported for Megalobrama amblycephala (Zhou et al., 2013) and salmonids (Walton and Cowey, 1982).
The poor inhibition of gluconeogenesis by dietary carbohydrates is considered one of the reasons for the glucose intolerance of carnivorous fish (Panserat et al., 2000). In our study, the levels of key gluconeogenesis enzymes, including GK, PK and HK, showed similar trends, and higher activities were all recorded in the high-starch group, while experimental fish fed a high-starch diet supplemented with phytosterol had results similar to those of the low-starch group. Moreover, the lowest hepatic GK level was found in fish fed a low-starch diet among the groups. The opposite trend was observed for hepatic gluconeogenesis enzymes, and relatively lower activities of PEK and PEPCK were both found in the low-starch group. The different results could be ascribed primarily to differences in dietary starch levels and dietary phytosterol supplementation levels. The regulation of the gluconeogenic pathway by dietary carbohydrate sources is still not consistent, and the different responses of fish to dietary carbohydrate sources should be further clarified. The muscle and hepatic glycogen contents of juvenile M. salmoides fed the experimental diets for 8 weeks were also recorded. Because of the core role of the liver in glycogen reservation, the hepatic glycogen content was significantly influenced by dietary treatment compared to the muscle glycogen content. With increasing dietary phytosterol content, hepatic glycogen decreased gradually, corresponding to changes in the activities of glucose metabolism-related enzymes and in the concentrations of plasma biochemical indices, which might indicate that supplemental phytosterols could promote glucose utilization and metabolism and alleviate the negative stimulation of continuous glycemia stress in M. salmoide fed a high-starch diet.
5 Conclusion
In summary, dietary phytosterol supplementation could enhance the growth performance, feed utilization, antioxidant status and glucose metabolism of juvenile largemouth bass (Micropterus salmoides) fed a high-starch diet. In addition, the present study revealed that dietary phytosterol supplementation plays a key role in ameliorating the negative stimulation of continuous glycemia stress induced by high starch. Therefore, further studies should focus on the relationships between phytosterol and glucose metabolism as well as determining the metabolic pathways involved under high starch stress to facilitate better dietary starch utilization to reduce the feed costs of M. salmoide.
Data availability statement
The original contributions presented in the study are included in the article/supplementary material. Further inquiries can be directed to the corresponding authors.
Ethics statement
The animal study was approved by Institutional Review Board of the Science Technology Department of Zhejiang Province. The study was conducted in accordance with the local legislation and institutional requirements.
Author contributions
QL: Conceptualization, Data curation, Formal Analysis, Investigation, Project administration, Writing – original draft. YH: Data curation, Formal Analysis, Investigation, Methodology, Writing – review & editing. NZ: Investigation, Methodology, Writing – review & editing. YB: Investigation, Software, Writing – review & editing. WS: Investigation, Software, Writing – review & editing. XC: Investigation, Validation, Writing – review & editing. GY: Investigation, Validation, Writing – review & editing. QM: Investigation, Validation, Writing – review & editing. RH: Investigation, Validation, Writing – review & editing. XD: Funding acquisition, Project administration, Supervision, Writing – review & editing. FZ: Data curation, Funding acquisition, Project administration, Resources, Supervision, Writing – review & editing.
Funding
The author(s) declare financial support was received for the research, authorship, and/or publication of this article. This study was funded by the Key Scientific and Technological Grant of Zhejiang for Breeding New Agricultural (Aquaculture) Varieties, Grant/Award Number: 2021C02069-2; the China Agriculture Research System, Grant/Award Number: CARS-46; and the Project of Zhejiang Provincial Department of Agriculture and Rural Affairs, Grant/Award Number: 2023SNJF074, 2024SNJF056.
Acknowledgments
We gratefully acknowledge the field staff of Zhejiang Fishery Extension Center for providing countless hours of feeding and collecting project-associated data.
Conflict of interest
The authors declare that the research was conducted in the absence of any commercial or financial relationships that could be construed as a potential conflict of interest.
Publisher’s note
All claims expressed in this article are solely those of the authors and do not necessarily represent those of their affiliated organizations, or those of the publisher, the editors and the reviewers. Any product that may be evaluated in this article, or claim that may be made by its manufacturer, is not guaranteed or endorsed by the publisher.
Abbreviations
PS, phytosterol; LS, low starch; HS, high starch; IBW, initial body weight; FBW, final body weight; FLW, final liver weight; FVW, final visceral weight; IBW, initial body weight; FBW, final body weight; FLW, final liver weight; FVW, final visceral weight; WG, weight gain; SGR, specific growth rate; FI, feed intake; FCR, feed conversion ratio; HSI, hepatosomatic index; VSI, visceral index; CF, condition factor; PER, protein efficiency ratio; IPFR, intraperitoneal fat ratio; TP, total protein; TCH, total cholesterol; TG, triglyceride; GLU, glucose; SOD, superoxide dismutase; CAT, catalase; ALT, alanine amiotransferase; AST, aspartate transaminase; MDA, malondialdehyde; GK, glucokinase; HK, hexokinase; PK, pyruvate kinase; PEK, phosphoglucokinase; PEPCK, phosphoenolpyruvate carboxykinase.
References
Borin-Crivellenti S., Crivellenti L. Z., de Oliveira F. R., Costa P. B., Alvarenga A. W. O., Rezende L. R., et al. (2021). Effect of phytosterols on reducing low-density lipoprotein cholesterol in dogs. Domest. Anim. Endocrinol. 76, e106610. doi: 10.1016/j.domaniend.2021.106610
Castex M., Lemaire P., Wabete N., Chim L. (2009). Effect of dietary probiotic Pediococcus acidilactici on antioxidant defences and oxidative stress status of shrimp Litopenaeus stylirostris. Aquacult. 294, 306–313. doi: 10.1016/j.aquaculture.2009.06.016
Chen P., Zhu Y. P., Wu X. F., Gu X., Xue M., Liang X. F., et al. (2023). Metabolic adaptation to high-starch diet in largemouth bass (Micropterus salmoides) was associated with the restoration of metabolic functions via inflammation, bile acid synthesis and energy metabolism. Brit. J. Nutr. 129, 381–394. doi: 10.1017/S0007114522001180
Cheng Y., Chen Y., Li J., Qu H., Zhou Y. (2020). Dietary β-sitosterol regulates serum lipid level and improves immune function, antioxidant status, and intestinal morphology in broilers. Poult. Sci. 99, 1400–1408. doi: 10.1016/j.psj.2019.10.025
Couto A., Kortner T. M., Penn M., Bakke A. M., Krogdahl Å., Oliva-Teles A., et al. (2014). Effects of dietary soy saponins and phytosterols on gilthead sea bream (Sparus aurata) during the on-growing period. Anim. Feed Sci. Technol. 198, 203–214. doi: 10.1016/j.anifeedsci.2014.09.005
Couto A., Kortner T. M., Penn M., Østby G., Bakke A. M., Krogdahl Å., et al. (2015). Saponins and phytosterols in diets for European sea bass (Dicentrarchus labrax) juveniles: effects on growth, intestinal morphology and physiology. Aquacult. Nutr. 21, 180–193. doi: 10.1111/anu.2015.21.issue-2
Ding X. Q., Yuan C. C., Huang Y. B., Jiang L., Qian L. C. (2021). Effects of phytosterol supplementation on growth performance, serum lipid, proinflammatory cytokines, intestinal morphology, and meat quality of white feather broilers. Poult. Sci. 100, e101096. doi: 10.1016/j.psj.2021.101096
Doornbos A. M. E., Meynen E. M., Duchateau G.S.M.J.E., van der Knaap H. C. M., Trautwein E. A. (2005). Intake occasion affects the serum cholesterol lowering of a plant sterol-enriched single dose yoghurt drink in mildly hypercholesterolemic subjects. Eur. J. Clin. Nutr. 60, 325–333. doi: 10.1038/sj.ejcn.1602318
Enes P., Peres H., Couto A., Oliva-Teles A. (2010). Growth performance and metabolic utilization of diets including starch, dextrin, maltose or glucose as carbohydrate source by gilthead sea bream (Sparus aurata) juveniles. Fish Physiol. Biochem. 36, 903–910. doi: 10.1007/s10695-009-9366-y
Fang Z. S., Gong Y., Han Z. H., Xie R. T., Li W. F., Zhang H. T., et al. (2024). Dietary sodium diacetate inclusion relieved hepatic glycogen deposition, oxidative stress, and intestinal microbial imbalance of largemouth bass (Micropterus salmoides) fed high dietary carbohydrate. Aquacult. 580, e740307. doi: 10.1016/j.aquaculture.2023.740307
Fırat Ö., Cogun H. Y., Yüzereroğlu T. A., Gök G., Fırat Ö., Kargin F., et al. (2011). A comparative study on the effects of a pesticide (cypermethrin) and two metals (copper, lead) to serum biochemistry of Nile tilapia, Oreochromis niloticus. Fish Physiol. Biochem. 37, 657–666. doi: 10.1007/s10695-011-9466-3
Guo J. L., Kuang W. M., Zhong Y. F., Zhou Y. L., Chen Y. J., Lin S. M. (2020). Effects of supplemental dietary bile acids on growth, liver function and immunity of juvenile largemouth bass (Micropterus salmoides) fed high-starch diet. Fish Shellfish Immunol. 97, 602–607. doi: 10.1016/j.fsi.2019.12.087
He Y., Tang Y., Xu N., Yao C., Gong Y., Yin Z., et al. (2022). Effects of supplemental phytosterol on growth performance, body composition, serum biochemical indexes and lipid metabolism of juvenile large yellow croaker (Larimichthys crocea) fed with high lipid diet. Aquacult. 551, e737889. doi: 10.1016/j.aquaculture.2022.737889
Hemre G. I., Mommsen T. P., Krogdahl A. (2002). Carbohydrates in fish nutrition: effects on growth, glucose metabolism and hepatic enzymes. Aquacult. Nutr. 8, 175–194. doi: 10.1046/j.1365-2095.2002.00200.x
Hou H. C., Ren A. Q., Yu L. X. B., Ma Z., Zhang Y., Liu Y., et al. (2023). An environmental impact assessment of largemouth bass (Micropterus salmoides) aquaculture in Hangzhou, China. Sustainability 15, e12368. doi: 10.3390/su151612368
Hu Q. L., Li S. S., Zhang Y. W., Zhuo Z., Feng J. (2017). Phytosterols on growth performance, antioxidant enzymes and intestinal morphology in weaned piglets. J. Sci. Food Agricult. 97, 4629–4634. doi: 10.1002/jsfa.8333
Huang X. L., Zhong L., Kang Q., Liu S., Feng Y., Geng Y., et al. (2021). A high starch diet alters the composition of the intestinal microbiota of largemouth bass. Front. Microbiol. 12, e696588. doi: 10.3389/fmicb.2021.696588
Ighodaro O. M., Akinloye O. A. (2018). First line defence antioxidants-superoxide dismutase (sod), catalase (cat) and glutathione peroxidase (gpx): their fundamental role in the entire antioxidant defence grid. Alexa. J. Med. 54, 287–293. doi: 10.1016/j.ajme.2017.09.001
Laraki L., Pelletier X., Mourot J., Debry G. (1993). Effects of dietary phytosterols on liver lipids and lipid metabolism enzymes. Ann. Nutr. Metab. 37, 129–133. doi: 10.1159/000177760
Li S. L., Sang C. Y., Wang A., Zhang J. C., Chen N. S. (2019). Effects of dietary carbohydrate sources on growth performance, glycogen accumulation, insulin signaling pathway and hepatic glucose metabolism in largemouth bass, Micropterus salmoides. Aquacult. 513, e734391. doi: 10.1016/j.aquaculture.2019.734391
Li X., Wei X., Guo X., Mi S. C., Hua X. M., Li N. Y., et al. (2020). Enhanced growth performance, muscle quality and liver health of largemouth bass (Micropterus salmoides) were related to dietary small peptides supplementation. Aquacult. Nutr. 26, 2169–2177. doi: 10.1111/anu.13155
Li X. Y., Zheng S. X., Ma X. K., Cheng K. M., Wu G. Y. (2021). Use of alternative protein sources for fishmeal replacement in the diet of largemouth bass (Micropterus salmoides). Part I: effects of poultry by-product meal and soybean meal on growth, feed utilization, and health. Amino Acids 53, 33–47. doi: 10.1007/s00726-020-02920-6
Liang H. L., Xu G. C., Xu P., Zhu J., Li S. L., Ren M. C., et al. (2022). Dietary histidine supplementation maintained amino acid homeostasis and reduced hepatic lipid accumulation of juvenile largemouth bass, Micropterus salmoides. Aquacult. Nutr. 2022, e4034922. doi: 10.1155/2022/4034922
Liland N. S., Espe M., Rosenlund G., Waagb R., Torstensen B. E. (2013). High levels of dietary phytosterols affect lipid metabolism and increase liver and plasma tag in Atlantic salmon (Salmo salar L.). Brit. J. Nutr. 110, 1–10. doi: 10.1017/S0007114513001347
Lin S. M., Shi C. M., Mu M. M., Chen Y. J., Luo L. (2018). Effect of high dietary starch levels on growth, hepatic glucose metabolism, oxidative status and immune response of juvenile largemouth bass, Micropterus salmoides. Fish Shellfish Immunol. 78, 121–126. doi: 10.1016/j.fsi.2018.04.046
Liu Y. J., Liu N., Wang A., Chen N. S., Li S. S. (2021). Resveratrol inclusion alleviated high-dietary-carbohydrate-induced glycogen deposition and immune response of largemouth bass, Micropterus salmoides. Brit. J. Nutr. 127, 1–33. doi: 10.1017/S0007114521000544
Lundstedt L. M., José F. B. M., Moraes G. (2004). Digestive enzymes and metabolic profile of Pseudoplatystoma corruscans (teleostei: siluriformes) in response to diet composition. Compar. Biochem. Physiol(B). 137, 331–339. doi: 10.1016/j.cbpc.2003.12.003
Ma H. J., Mou M. M., Pu D. C., Lin S. M., Chen Y. J., Luo L., et al. (2019). Effect of dietary starch level on growth, metabolism enzyme and oxidative status of juvenile largemouth bass, Micropterus salmoides. Aquacult. 498, 482–487. doi: 10.1016/j.aquaculture.2018.07.039
Marineli R. D. S., Marques A. Y. C., Furlan C. P. B., Maróstica M. R. Jr. (2012). Antioxidant effects of the combination of conjugated linoleic acid and phytosterol supplementation in sprague–dawley rats. Food Res. Int. 49, 487–493. doi: 10.1016/j.foodres.2012.07.022
Matvienko O. A., Lewis D. S., Swanson M., Arndt B., Rainwater D. L., Stewart J., et al. (2002). A single daily dose of soybean phytosterols in ground beef decreases serum total cholesterol and LDL cholesterol in young, mildly hypercholesterolemic men. Am. J. Clin. Nutr. 76, 57–64. doi: 10.1093/ajcn/76.1.57
Naji T., Amadou I., Zhao R. Y. (2014). Effects of phytosterol in feed on growth and related gene expression in muscles of broiler chickens. Trop. J. Pharm. Res. 13, 9–16. doi: 10.4314/tjpr.v13i1.2
Panserat S., Médale F., Blin C., Brèque J., Kaushik S. J. (2000). Hepatic glucokinase is induced by dietary carbohydrates in rainbow trout, gilthead seabream, and common carp. Regulat. Integrat. Compar. Physiol. 278, 1164–1170. doi: 10.1152/ajpregu.2000.278.5.R1164
Reyes-Becerril M., Angulo C., Cosío-Aviles L., López M. G., Calvo-Gómez O. (2022). Cylindropuntia cholla aqueous root rich in phytosterols enhanced immune response and antimicrobial activity in tilapia Oreochromis niloticus leukocytes. Fish Shellfish Immunol. 131, 408–418. doi: 10.1016/j.fsi.2022.10.028
Shadrack R. S., Manabu I., Koshio S., Yokoyama S., Zhang Y. K., Mzengereza K., et al. (2022). Effects of single and mixture probiotic supplements on growth, digestive activity, antioxidative status, immune and growth related genes, and stress response of juvenile red sea bream (Pagrus Major). Aquacult. Nutr. 2022, e8968494. doi: 10.1155/2022/8968494
Tan Q. S., Wang F., Xie S. Q., Zhu X. M., Lei W., Shen J. Z. (2010). Effect of high dietary starch levels on the growth performance, blood chemistry and body composition of gibel carp (Carassius auratus var. Gibelio). Aquacult. Res. 40, 1011–1018. doi: 10.1111/j.1365-2109.2009.02184.x
Trautwein E. A., Demonty I. (2007). Phytosterols: natural compounds with established and emerging health benefits. Oilseeds Crops fats Lipids 14, 259–266. doi: 10.1051/ocl.2007.0145
Tsikas D. (2017). Assessment of lipid peroxidation by measuring malondialdehyde (MDA) and relatives in biological samples: analytical and biological challenges. Anal. Biochem. 524, 13–30. doi: 10.1016/j.ab.2016.10.021
Walton M. J., Cowey C. B. (1982). Aspects of intermediary metabolism in salmonid fish. Compar. Biochem. Physiol. (B) 73, 59–79. doi: 10.1016/0305-0491(82)90201-2
Wang Y. Y., Xie S. M., Nie Z. J., Li Q. J., Xu G. C. (2020). Optimum feeding frequency of juvenile largemouth bass (Micropterus salmoides) reared in in-pond raceway recirculating culture system. Fish Physiol. Biochem. 46, 2197–2212. doi: 10.1007/s10695-020-00866-w
Wu C. L., Ye J. Y., Gao J. E., Chen L., Lu Z. B. (2016). The effects of dietary carbohydrate on the growth, antioxidant capacities, innate immune responses and pathogen resistance of juvenile black carp Mylopharyngodon piceus. Fish Shellfish Immunol. 49, 132–142. doi: 10.1016/j.fsi.2015.12.030
Wu P., Chen Y. P., When C., Tang Z. G., Zhou Y. M. (2012). Effects of different phytosterols on growth and meat quality of ducks. J. CN cereals oils Assoc. 1, 75–79. doi: 10.3969/j.issn.1003-0174.2012.01.017
Xia Y., Wang G. J., Yu E. M., Tian J. J., Li Z. F., Zhang K. (2022). Addition of berberine to formulated feed changes the glucose utilisation, intestinal microbiota and serum metabolites of largemouth bass (Micropterus salmoides). Aquacult. Rep. 23, e101018. doi: 10.1016/j.aqrep.2022.101018
Xie X. M., Xiao N., He J. B. (2015). Physiological function ofphytosterol and its application. Anim. Husbandry Feed Sci. 7, 67–69, 105. Available at: http://www.hznet.com.cn/asset/detail$exname?id=203118192087.
Yu H. H., Zhang L. L., Chen P., Liang X. F., Cao A. Z., Han J., et al. (2019). Dietary bile acids enhance growth, and alleviate hepatic fibrosis induced by a high starch diet via AKT/FOXO1 and cAMP/AMPK/SREBP1 pathway in Micropterus salmoides. Front. Physiol. 10, e1430. doi: 10.3389/fphys.2019.01430
Zare M., Esmaeili N., Paolacci S., Stejskal V. (2023). Nettle (Urtica dioica) additive as a growth promoter and Immune stimulator in fish. Aquacult. Nutr. 2023, e8261473. doi: 10.1155/2023/8261473
Zhang Y. W., Jian-Jun R., Wen C., Liu W. B., Zhou Y. M. (2012). Effects of phytosterol on growth performance, plasma biochemical parameters and antioxidant parameters in hepatopancreas of bluntnose black bream Megalobrama amblycephala. Fisheries Sci. 21, 91. doi: 10.16378/j.cnki.1003-1111.2012.04.004
Zhang Y. M., Xie S. W., Guo T. Y., Liu Z. L., Fang H. H., Zheng L., et al. (2020). High dietary starch inclusion impairs growth and antioxidant status, and alters liver organization and intestinal microbiota in largemouth bass Micropterus salmoides. Aquacult. Nutr. 26, 1806–1821. doi: 10.1111/anu.13131
Zhao Y. R., Chen Y. P., Cheng Y. F., Qu H. M., Li J., Wen C., et al. (2019). Effects of dietary phytosterols on growth performance, antioxidant status, and meat quality in partridge shank chickens. Poult. Sci. 98, 3715–3721. doi: 10.3382/ps/pez059
Zhou C. P., Ge X. P., Liu B., Xie J., Miao L. H. (2013). Effect of high dietary carbohydrate on the growth performance and physiological responses of juvenile wuchang bream, Megalobrama amblycephala. Asian Austral. J. Anim. Sci. 26, 1598–1608. doi: 10.5713/ajas.2012.12659
Keywords: phytosterol, Micropterus salmoides, high-starch diet, growth performance, feed utilization, antioxidant status, glucose metabolism
Citation: Liang Q, Huang Y, Zhu N, Bei Y, Shi W, Chen X, Yao G, Meng Q, He R, Ding X and Zhou F (2024) Phytosterol supplementation enhances the growth performance, feed utilization, antioxidant status and glucose metabolism of juvenile largemouth bass (Micropterus salmoides) fed a high-starch diet. Front. Mar. Sci. 11:1403563. doi: 10.3389/fmars.2024.1403563
Received: 19 March 2024; Accepted: 23 April 2024;
Published: 13 May 2024.
Edited by:
Lei Wang, Anhui Normal University, ChinaReviewed by:
Peng Tan, Marine Fishery Institute of Zhejiang Province, ChinaLiwei Liu, Huazhong Agricultural University, China
Copyright © 2024 Liang, Huang, Zhu, Bei, Shi, Chen, Yao, Meng, He, Ding and Zhou. This is an open-access article distributed under the terms of the Creative Commons Attribution License (CC BY). The use, distribution or reproduction in other forums is permitted, provided the original author(s) and the copyright owner(s) are credited and that the original publication in this journal is cited, in accordance with accepted academic practice. No use, distribution or reproduction is permitted which does not comply with these terms.
*Correspondence: Xueyan Ding, dingxy_sc@sina.com; Fan Zhou, zhoufan0302@126.com
†These authors have contributed equally to this work