Solid-state fermentation converts rice bran into a high-protein feed ingredient for Penaeus monodon
- Institute of Aquaculture, College of Fisheries and Ocean Sciences, University of the Philippines Visayas, Miagao, Iloilo, Philippines
Fermented rice bran (FRB) was evaluated as an alternative protein source to soybean meal (SM) in practical diets for juvenile black tiger shrimp, Penaeus monodon. This feed ingredient was tested in a feeding trial to replace soybean meal in P. monodon diets at 0% (T0), 12.5% (T12.5), 25% (T25), 37.5% (T37.5), and 50% (T50). Five iso-nitrogenous and iso-caloric experimental diets containing 44% crude protein were fed to groups of juvenile shrimp (0.47 ± 0.002 g) randomly assigned to twenty 60-l rectangular tanks equipped with a recirculating seawater system. Each dietary treatment was run in 4 replicates, and the feeding trial lasted 50 days. Results show significant improvement in weight gain, specific growth rate, and protein efficiency ratio in treatment T12.5 and T25. Treatments with higher levels of SM replacement with FRB exhibited similar growth indices to those of the control group. Polynomial regression analysis indicates that the optimum replacement of soybean meal with FRB for optimum growth is 21.08%. The apparent dry matter and protein digestibility coefficients of FRB are 83.05 ± 0.02% and 87.20 ± 0.30%, respectively. There were no significant differences in the whole-body composition (dry matter, protein, lipid, ash) among treatments of shrimp fed with FRB replacement. The data suggest that FRB replacement of dietary soybean meal is feasible at 50% without affecting the growth performance but may promote growth at 21.08% replacement of P. monodon.
1 Introduction
The global aquaculture production of giant black tiger shrimp (Penaeus monodon) in 2021 was 695,674 metric tons, valued at $ 5.9 billion, with more than 99% of production coming from Asian countries (FAOSTAT, 2024). The intensive farming of P. monodon in Asia has experienced sluggish growth at an annual rate of 0.9% in the past ten years (FAOSTAT, 2024). Similar to other Asian countries, the production of shrimp in the Philippines is declining, with an annual growth rate of -4.8% (PSA, 2024). Aside from diseases, the major constraint of shrimp aquaculture is feed cost. Feed ingredients used in commercial giant black tiger shrimp diets in many developing countries in Asia are mostly imported.
Among the imported feed ingredients, soybean meal (SBM) is the most important feed-protein source used in shrimp feeds (Brown et al., 2008). However, livestock and aquaculture industries compete in the use of SBM, and this has resulted in an increase in prices and erratic supply (Traifalgar et al., 2019). Since shrimp feed accounts for more than 50% of production cost (Hung and Quy, 2013), there is currently great interest in reducing feed costs using locally available feed ingredients such as rice bran.
One locally available feed ingredient is rice bran, which is cheap and available in large quantities. This material is produced as a by-product of the rice milling process and is mainly used as an energy source in animal feed (Phongthai et al., 2017). The Philippines is the 7th largest rice producer in the world and contributes 2.5% of global rice production (World Agricultural Production, 2024). Since rice bran accounts for about 8 to 11% of the grain, approximately 87 million metric tons are produced annually and could be a cheaper source of feed protein in shrimp diets (FAO, 2023). However, rice products are not normally used in shrimp feeds because they are similarly priced with wheat products but have no feed-binding properties (Akiyama et al., 1992). The limitation of its use is also attributed to its high fiber content (12.4-27.8%), low protein (7.8%), and the presence of anti-nutritional factors (Luh, 1991; Tillman et al., 2005; Hertrampf, 2006).
The fungus Trichoderma is renowned for its ability to colonize diverse ecological niches, including soil, roots, and leaves, and is particularly noted for its secretion of substantial quantities of cellulases. This fungus has been documented to be a fast colonizer of cellulosic substrates and does not produce aflatoxins (Ahmed et al., 2009; Bulgari et al., 2023). Furthermore, it is known that this fungus can grow and utilize cellulosic rice stalks and rice milling by-products as substrates and convert them into fungal biomass. Additionally, Trichoderma harzianum has been previously reported to be used in the fermentation of rice by-products, such as rice husk and rice polishing (Ahmed et al., 2017; Sala et al., 2019).
Several studies have been conducted to improve the quality of rice bran and increase its utilization as a feed ingredient (Schmidt and Furlong, 2012; Kang et al., 2015; Supriyati et al., 2015; Hong et al., 2016). Among this technique is biomass transformation through solid-state fermentation (SSF). Fermentation of rice bran increases its nutrient availability through changes arising from microorganisms’ metabolic activity (da Silveirai and Furlong, 2007; Jang and Yang, 2008), increases protein and soluble sugars, and reduces complex carbohydrates (Iyayi and Aderolu, 2004). However, to this date, there are no published reports on the use of fermented rice bran (FRB) in shrimp feeds, and information regarding feed value and biological testing in aquatic animals is limited. In the present study, we evaluated the feed value of SSF rice bran as a replacement for soybean meal in the diet of juvenile P. monodon.
2 Materials and method
2.1 Rice bran fermentation
2.1.1 Microbial inoculum
Fungus T. harzianum was sourced from the Bureau of Soils and Water Management, Department of Agriculture Regional Field Office 6, Iloilo City, Philippines. The fungus was inoculated on Petri dishes containing potato dextrose agar medium (PDA), incubated at 30°C for seven days, and stored at four °C. Spores were collected aseptically, and the count was determined by serial dilution followed by the spread plating method. Spore count was expressed as colony-forming units (CFU) per milliliter.
2.1.2 Fermentation
Freshly milled rice bran was obtained from the Iloilo Rice Processing Complex in Pototan, Iloilo, Philippines. Broken rice and rice hull were separated from the bran using a 0.4 mm sieve and were kept at -20 ˚C until use. Rice bran was subjected to a solid-state fermentation system as previously described (Schmidt and Furlong, 2012). Trays (15 cm x 10 cm x 3.5 cm) containing 2 cm layers of rice bran portions (sterilized at 121°C for 15 min) were moistened with nutrient solution (0.8 g/l ammonium chloride). T. harzianum spores were added to an initial concentration of 3 x 10 6 spores/g of bran. Sterile distilled water was added to the medium to adjust the humidity to 50%. The trays were placed in a fermentation chamber composed of a plastic-coated insulated rectangular metal box with a dimension of 1.0 x 0.5 x 1.5 m with a temperature of 30 ˚C and relative humidity of 60%. After 96 hours of incubation, the fermented biomass was sterilized, oven-dried at 60°C, ground, sieved in 0.20 mm, and stored at -20°C.
2.2 Animals and experimental design
The experiment was conducted at the hatchery complex of the Institute of Aquaculture (IA), College of Fisheries and Ocean Sciences (CFOS), University of the Philippines Visayas (UPV) in Miagao, Iloilo, Philippines. Good quality and disease-free P. monodon post larvae (PL 15) were obtained from a private hatchery in Guimbal, Iloilo, Philippines. Samples were submitted to the Fish Health Section of the Southeast Asian Fisheries Development Center (SEAFDEC) Aquaculture Department for PCR analysis. The post larvae were acclimated and stocked in a 50-ton canvass pond for 30 days with a stocking density of 100 pieces/m3. Prior to stocking, tilapia “green water” was inoculated from the adjacent tank, and aeration was provided. Shrimp were fed with live artemia for seven days and a commercial diet at a feeding rate of 25% of total body weight three times per day. Feeding trays were used to monitor the growth and health of shrimp. A commercial probiotic, BZT ® Aquaculture (Lactobacillus plantarum, Bacillus subtilis, Bacillus licheniformis, and Saccharomyces cerevisiae), was applied once a week at one ppm to control ammonia, nitrate, and nitrite levels. No water exchange was done during the nursery phase. Water parameters were monitored regularly, and optimum levels were maintained. At the end of the nursery phase, juveniles were collected using a scoop net and transferred to the experimental set-up.
The juveniles (0.47 ± 0.002 g) were randomly distributed into twenty (20) units of 60-L plastic tanks with fifteen shrimp per tank and were acclimated for seven days. This set-up comprised five dietary treatments in four replicates arranged in a complete randomized design (CRD). Three artificial shelters fabricated using plastic sticks were placed in each tank to reduce cannibalism. The set-up ran on a recirculating system, and aeration was provided. Water temperature, salinity, dissolved oxygen (DO), and pH were monitored daily. Ammonia, nitrite, and nitrate were monitored two times per week using test kits (API Saltwater Master Test Kit, MARS Fishcare, USA). All of these water quality parameters were maintained at the optimum levels throughout the experimental period.
2.3 Experimental diet and feeding
Five isonitrogenous diets (44% protein and 12.4% lipid) were formulated by replacing 0,12.5, 25, 37.5, and 50% of soybean meal weight with FRB in the P. monodon diet. The composition of the experimental diets is shown in Table 1. Diet’s proximate composition analysis was determined following the official methods stipulated in AOAC (1990).
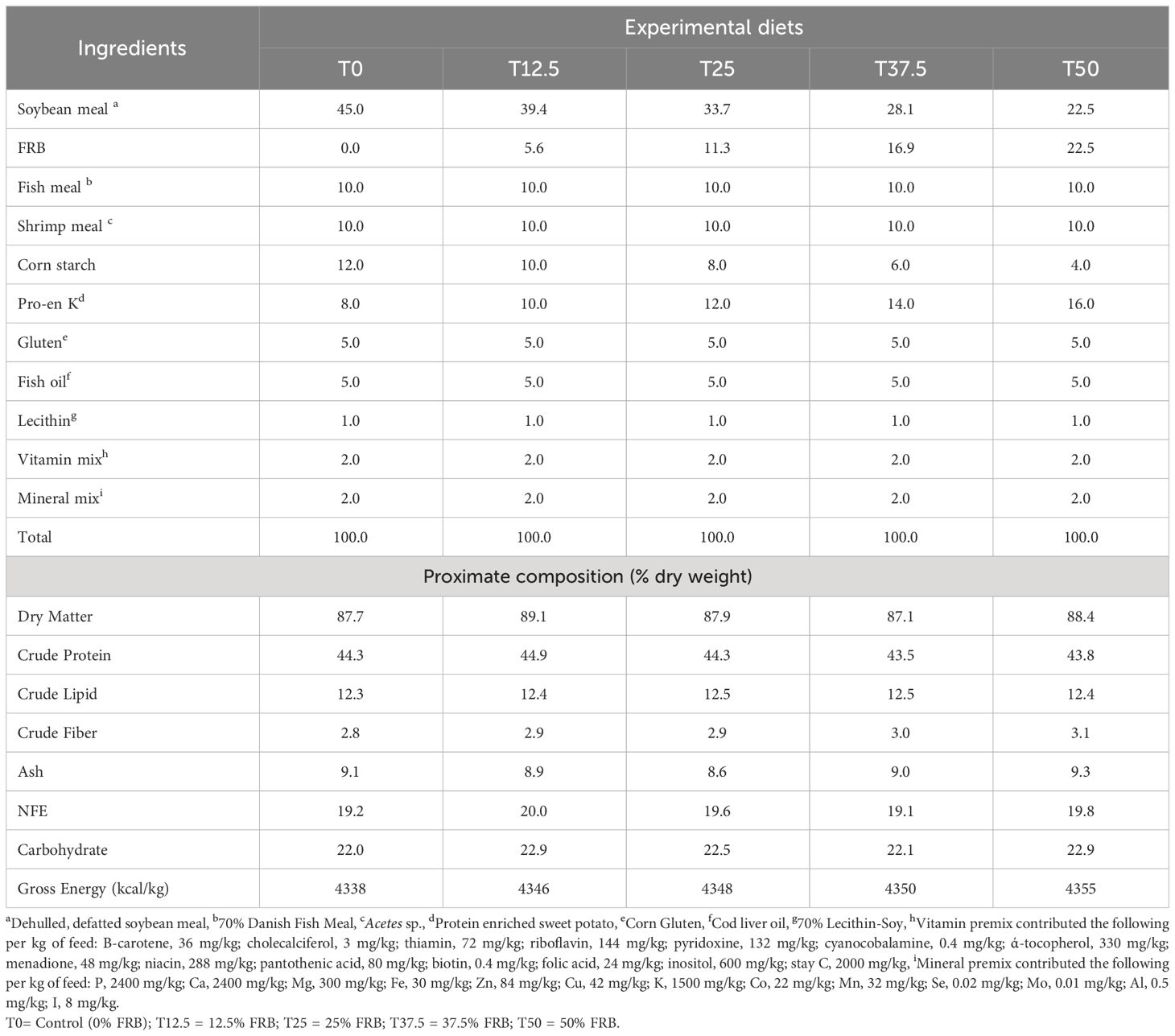
Table 1 Feed formulation (%) and proximate composition (% dry weight) of different experimental diets.
Once all of the dry ingredients were mixed by hand, soy lecithin and fish oil were added. Water was then gradually added (500 ml/kg) until the resulting dough could be easily extruded. The moist mixture was pelleted in a 2-mm diameter meat grinder. The 10 cm long “spaghetti-like” strands were oven-dried at 60°C for 18-24 hours. After drying, strands were broken, sieved to the appropriate size, packed in sealed plastic bags, and stored at -20°C until use.
Shrimp were fed ad libitum three times per day (8:00 AM, 12:00 NN, and 4:00 PM) for 50 days. Excess feeds were siphoned, dried, weighed every morning, and subtracted from the daily feed intake.
2.4 Evaluation of growth performance
The weight of shrimp was recorded at 10-day intervals for 50 days. At the end of the experiment, growth performance was evaluated and calculated by following these equations:
At the conclusion of the feeding trial, all shrimp samples from each tank were pooled, anesthetized at cold temperature, freeze-dried, and ground for approximate analyses of the whole-body composition following the standard methods (AOAC, 1990). Nutrient retention of P. monodon was calculated by the following equation:
2.5 Ingredient digestibility
Apparent digestibility coefficients for dry matter (ADMD) and crude protein (APD) of FRB as feed ingredient were measured using 1% chromium oxide (Cr2O3) as an inert marker. The method described by Cho et al. (1982) and Bautista-Teruel et al. (2003) was adapted using a combination of a reference diet to test ingredients in a proportion of 70:30 (Table 2).
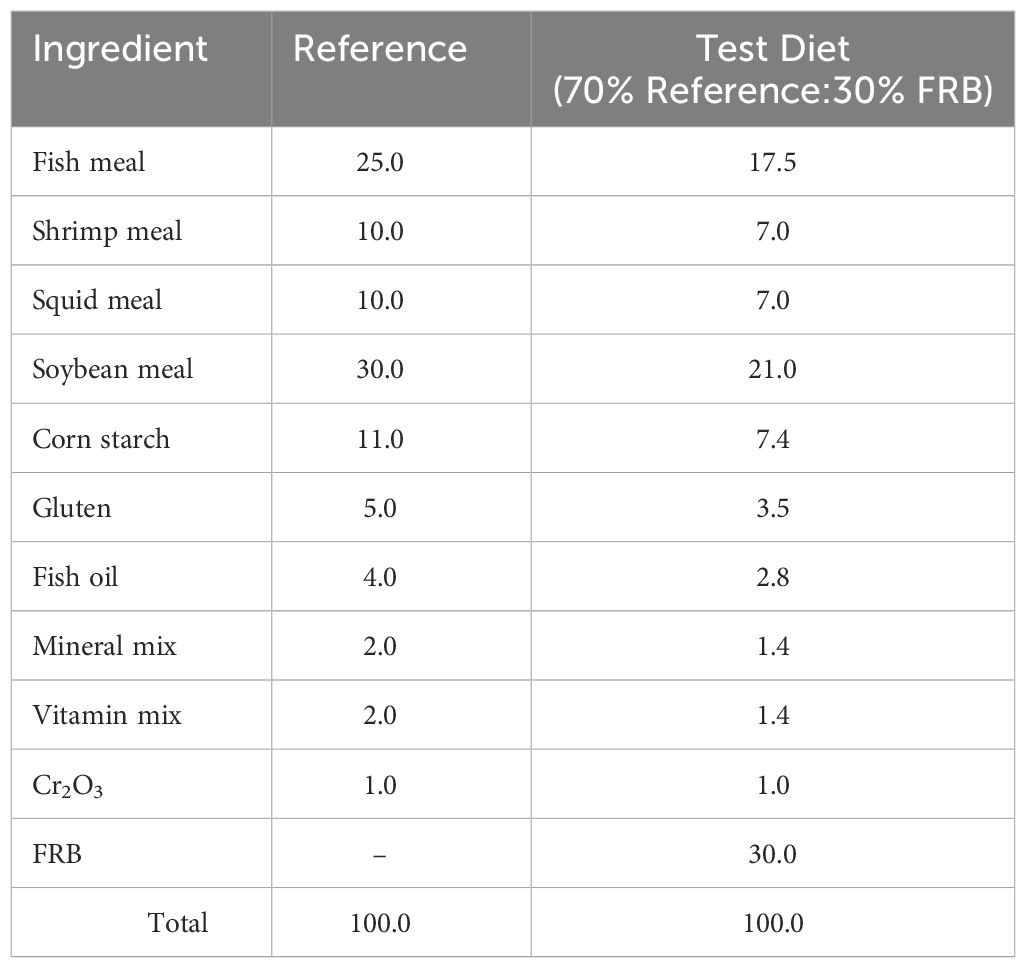
Table 2 Composition of reference and test diets for in vivo digestibility experiment in shrimp, P. monodon juveniles (g/100g feed).
In the digestibility assessment of the test ingredient, 25 shrimp were stocked in 250-l conical fiberglass tanks, with each dietary treatment run in triplicate. The experimental set-up was a flow-through culture system equipped with continuous aeration. Water temperature and salinity were maintained at 24–28°C and 30–34 ppt, respectively. Prior to the digestibility experiment, the shrimp were acclimated for seven days. The shrimp were fed with experimental diets ad libitum three times daily. One hour after feeding, uneaten feed and feces were removed, and fecal materials from the collection chamber were collected two hours after feeding. Collected feces were gently rinsed with distilled water, damp dried on filter paper, and oven-dried at 60°C. The dried feces were ground to a fine and homogeneous powder and stored in a -20°C freezer until analyzed.
Chromic oxide was analyzed in the feces and two diets using Ultraviolet-Visible-Near Infrared (UV-VIS-NIR) (Furukawa, 1966; Divakaran et al., 2002). Crude protein in the feces and two diets were analyzed following the official method stipulated in AOAC (1990).
Dry matter and protein apparent digestibility coefficients (ADCs) of diets were calculated using the following equation (Cho, 1979):
where: Cdiet = % chromic oxide in diet,
Ndiet = % nutrient in diet,
Nfeces = % nutrient in feces,
Cfeces = % chromic oxide in feces
ADCs of ingredients were calculated using the equation (Bureau and Hua, 2006):
where: ADCtestdiet = Apparent Digestibility Coefficient of test diet,
ADCref.diet = Apparent Digestibility Coefficient of reference diet,
Nref (as is) = nutrient in the reference diet,
Ningr (as is) = nutrient in the test diet
2.6 Proximate composition analysis
The FRB proximate composition analysis was determined by following the official methods stipulated in AOAC (1990). Crude protein was determined by the Kjeldahl method (Foss Tecator Digestion and Foss Kjeltec 8200 Auto Distillation Unit), while crude lipid was determined by Soxhlet extraction (Foss Soxtec 2050 Automatic System). Moisture was determined by a moisture analyzer (Mettler Toledo Halogen), and crude fiber was determined by the ceramic fiber filter method (Foss Fibertec 2010 System). The ash content was analyzed by furnace combustion (AOAC, 1984). Nitrogen-free extract (NFE) was calculated (Aksnes and Opstvedt, 1998), and NFE plus fiber is expressed as the total carbohydrate content. The gross energy of the diets and feces was also calculated (NRC, 2011).
2.7 Amino acid analysis
The FRB, experimental diets, and shrimp tail muscle amino acid profile were analyzed using Shimadzu High-Performance Liquid Chromatograph LC-10A/C-R7A Amino Acid Analysis System following the method detailed in AOAC Official Method 994.12 and Llames and Fontaine (1994) at the Nutrition Laboratory, IA, CFOS, UPV. The Chemical Score was calculated based on the methodologies outlined by Traifalgar et al. (2019) and Peñaflorida (1989) utilizing the essential amino acid requirements of P. monodon. The formula is presented below.
The Chemical Score Index (CSI) was the score of the lowest essential amino acid. The Essential Amino Acid Index (EAAI) of the feedstuffs was determined using the following formula:
where: aa 1 = the A/E (essential amino acid/total essential amino acid) ratio in the feed
AA1 = the A/E ratio in shrimp
n = number of essential amino acids
The EAAI was patterned after the formula for fish nutrition research (Castell and Tiews, 1980), with whole egg as the reference protein. In this study, however, whole juvenile P. monodon was used as the reference protein (Deshimaru and Shigeno, 1972).
2.8 Statistical analysis
The results of three replicate samples were analyzed by one-way analysis of variance (ANOVA) using IBM SPSS version 26. Differences between treatments were evaluated by Tukey’s test. In the case of two replicate samples, the independent samples t-test was used to analyze the means. Values were considered statistically significant at P< 0.05.
3 Results
3.1 Proximate composition and amino acid profile of rice bran, FRB, and SBM
After fermentation, rice bran crude protein and crude lipid increased by 169.2% (12.7 to 34.2%) and 40.3% (14.4 to 20.2%), respectively (Table 3). Conversely, rice bran crude fiber, ash, and NFE decreased by 87.1% (16.3 to 2.1%), 82.3% (12.4 to 2.2%), and 9.9% (34.4 to 31.0%), respectively. The proximate analyses of dehulled and defatted soybean meal used in diet formulation showed 12.0% moisture, 48.0% crude protein, 3.1% crude lipid, 3.0% crude fiber, 6.3% ash, and 27.6% NFE.
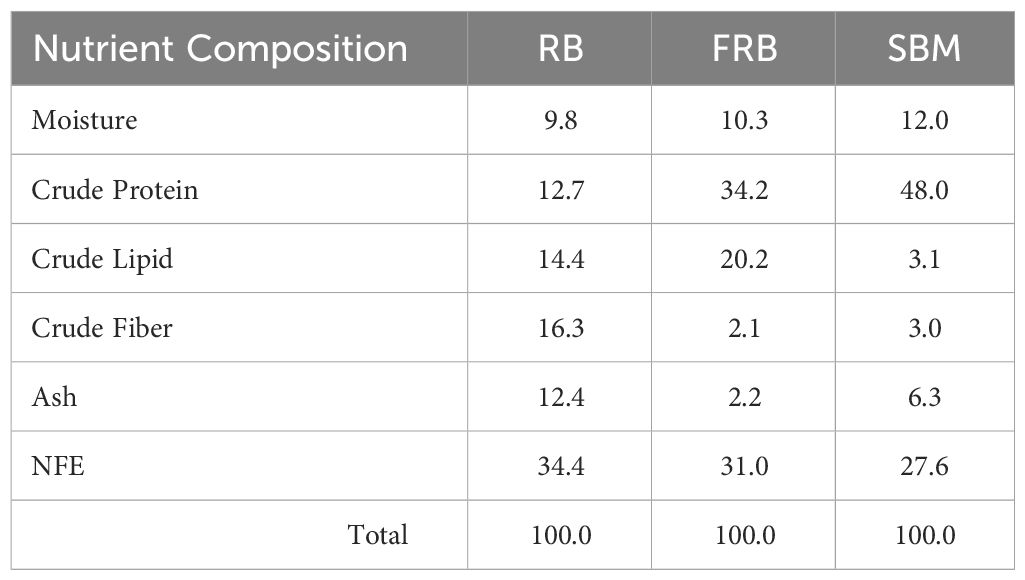
Table 3 Proximate composition (%) of rice bran (RB), fermented rice bran (FRB), and soybean meal (SBM).
Generally, there was an increase in the amount of amino acid in FRB compared to unfermented rice bran except for tryptophan (Table 4). All known essential amino acids for shrimp were found present in the FRB. The EAAI of FRB was 85.98, and the CSI was 25.20, with tryptophan as the limiting amino acid (Table 5). Using EAAI, FRB was rated as a good-quality protein material.
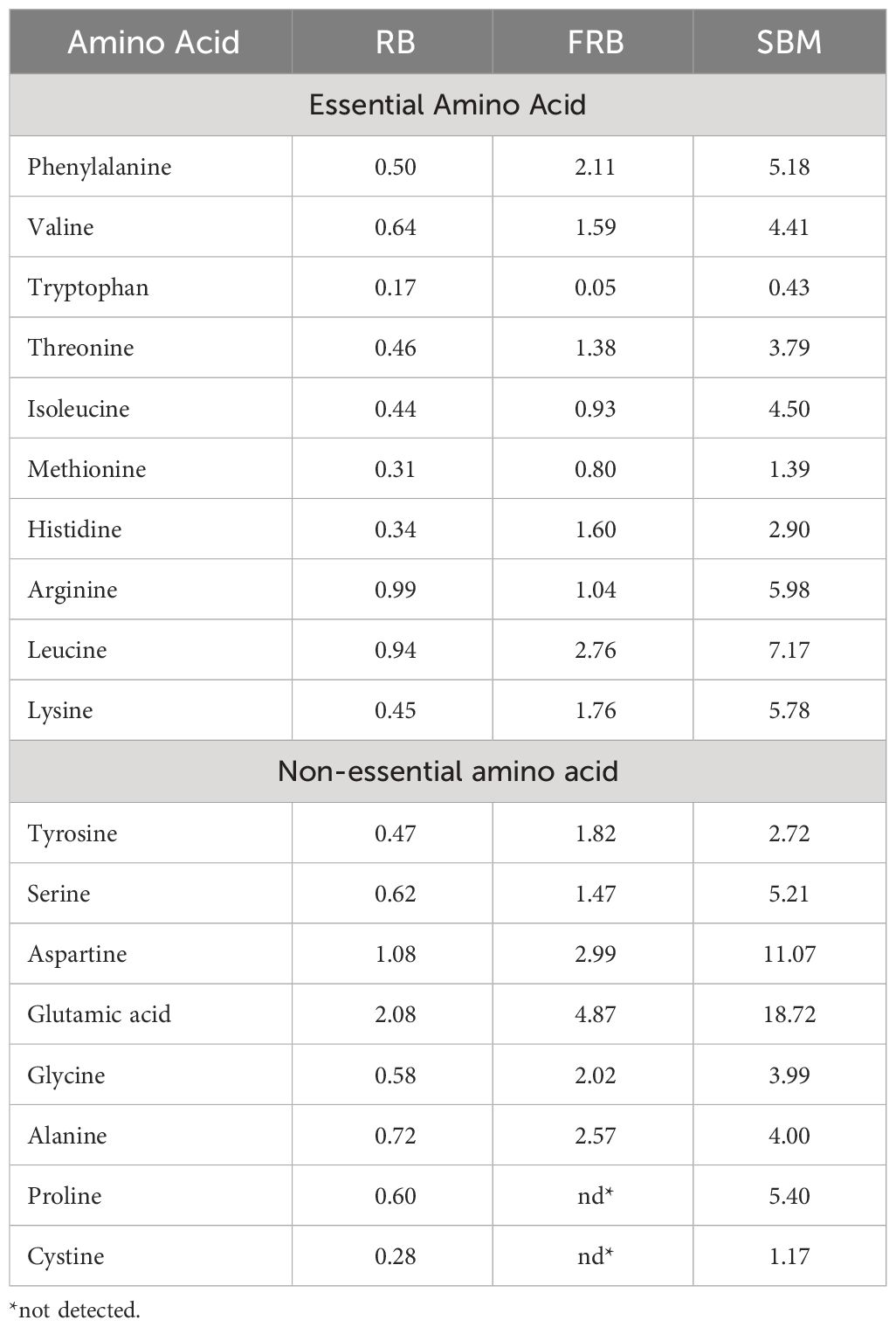
Table 4 Amino acid profile (% AA in protein) of rice bran (RB), fermented rice bran (FRB), and soybean meal (SBM).
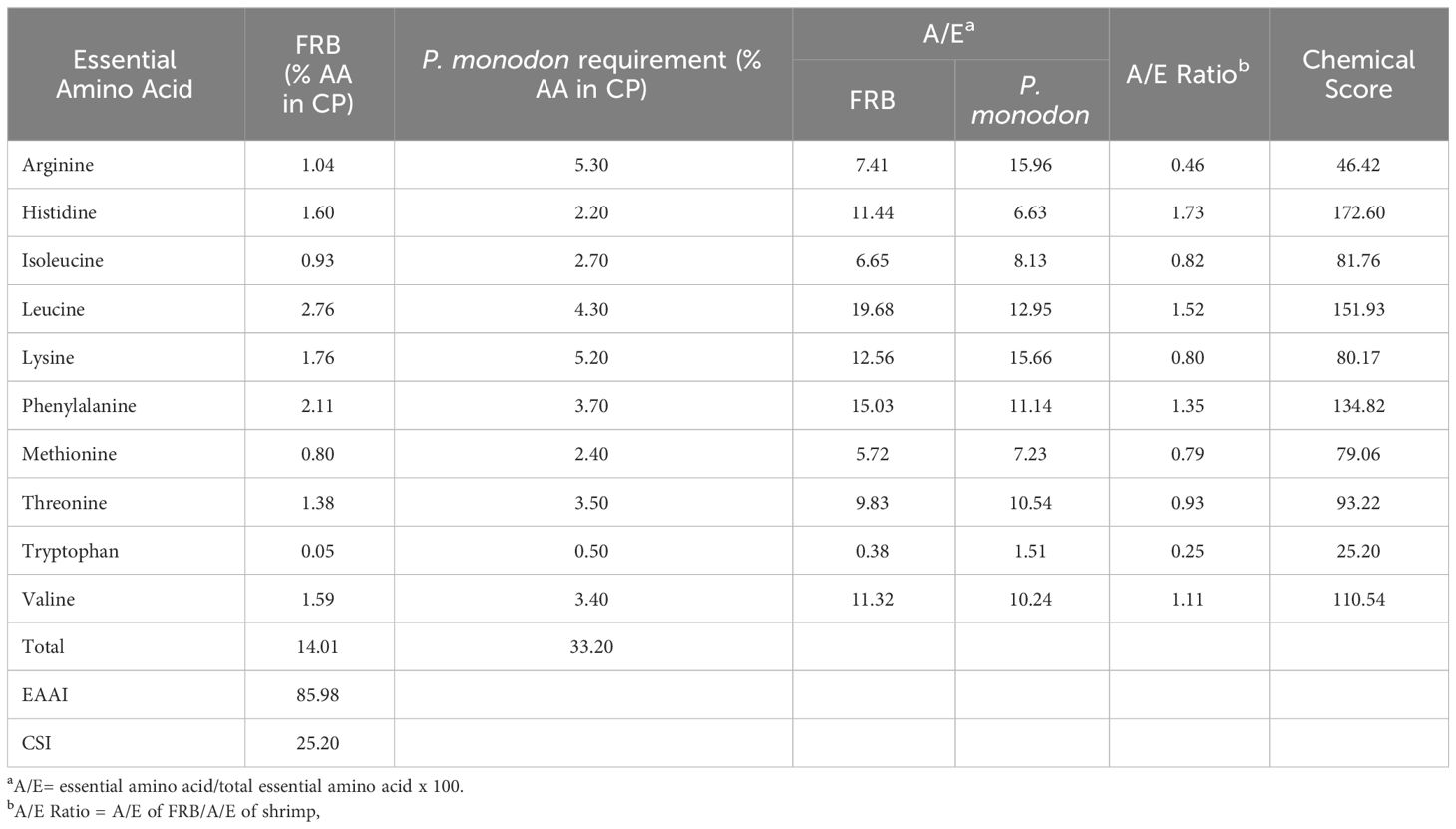
Table 5 Essential Amino Acid Index (EAAI) and Chemical Score Index (CSI) of fermented rice bran (FRB).
3.2 Apparent digestibility coefficients
The apparent dry matter (ADMD), protein (APD), and ingredient (ADI) digestibility coefficients of FRB by P. monodon are presented in Table 6. The FRB was found to be highly digestible with ADMD and APD coefficients at 83.05 ± 0.02% and 87.20 ± 0.30%, respectively. ADI coefficients were also high at 85.19 ± 0.08% in dry matter and 90.67 ± 0.78% in protein. Generally, the apparent digestibility coefficients of FRB were comparable to soybean meal and higher than unfermented rice bran.
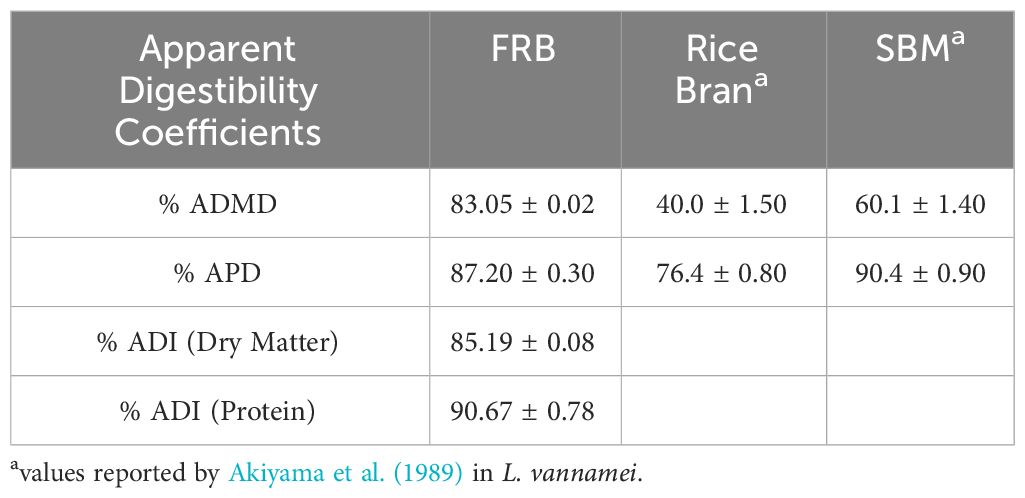
Table 6 Apparent digestibility coefficients (ADC) for dry matter, protein, and ingredients of fermented rice bran (FRB) by P. monodon.
3.3 Amino acids composition of experimental diets
The amino acid profile of the experimental diets, as detailed in Table 7, adequately meets the essential amino acid requirements of P. monodon. This means that the composition of amino acids present in the diets sufficiently fulfills the nutritional needs of P. monodon.
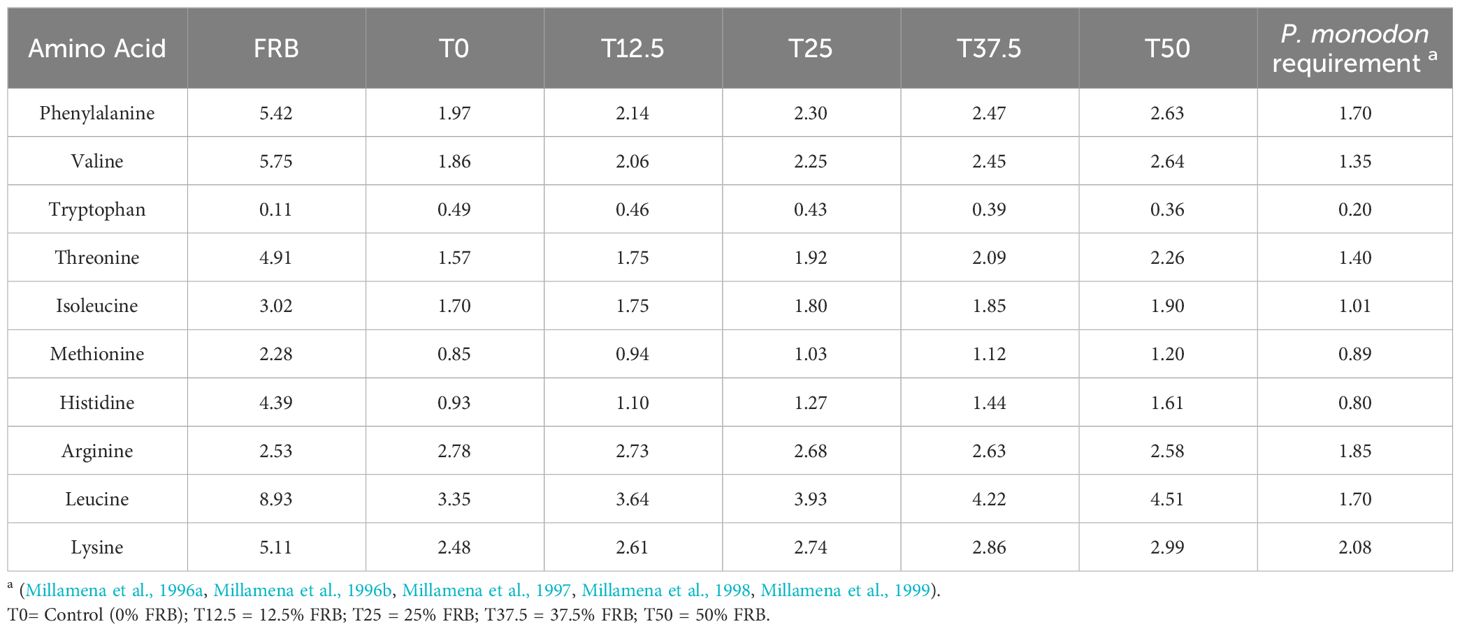
Table 7 Essential amino acid composition (g/100 g sample) of experimental diets with comparison to P. monodon requirement.
3.4 Growth performance of P. monodon
Significant differences (P< 0.05) were observed among the treatments in the growth parameters of P. monodon (Table 8). In terms of weight gain (%) and SGR, T25 was significantly higher (P< 0.05) than T0 but not significantly higher (P > 0.05) than T12.5. PER was significantly higher (P< 0.05) in T12.5 and T25 among treatments. Conversely, FCR was significantly lower in the same treatments compared to T0, T37.5, and T50. The DFI was highest in T25 at 30.7 ± 0.18 mg/day. There were no significant differences (P > 0.05) in survival among treatments. Generally, 0% and 50% replacement levels were observed to be significantly similar in terms of weight gain, SGR, PER, FCR, DFI, and survival.
The second-order polynomial regression analysis of weight gain suggests an FRB replacement level of 21.08% would provide the maximum growth for P. monodon juvenile (Figure 1).
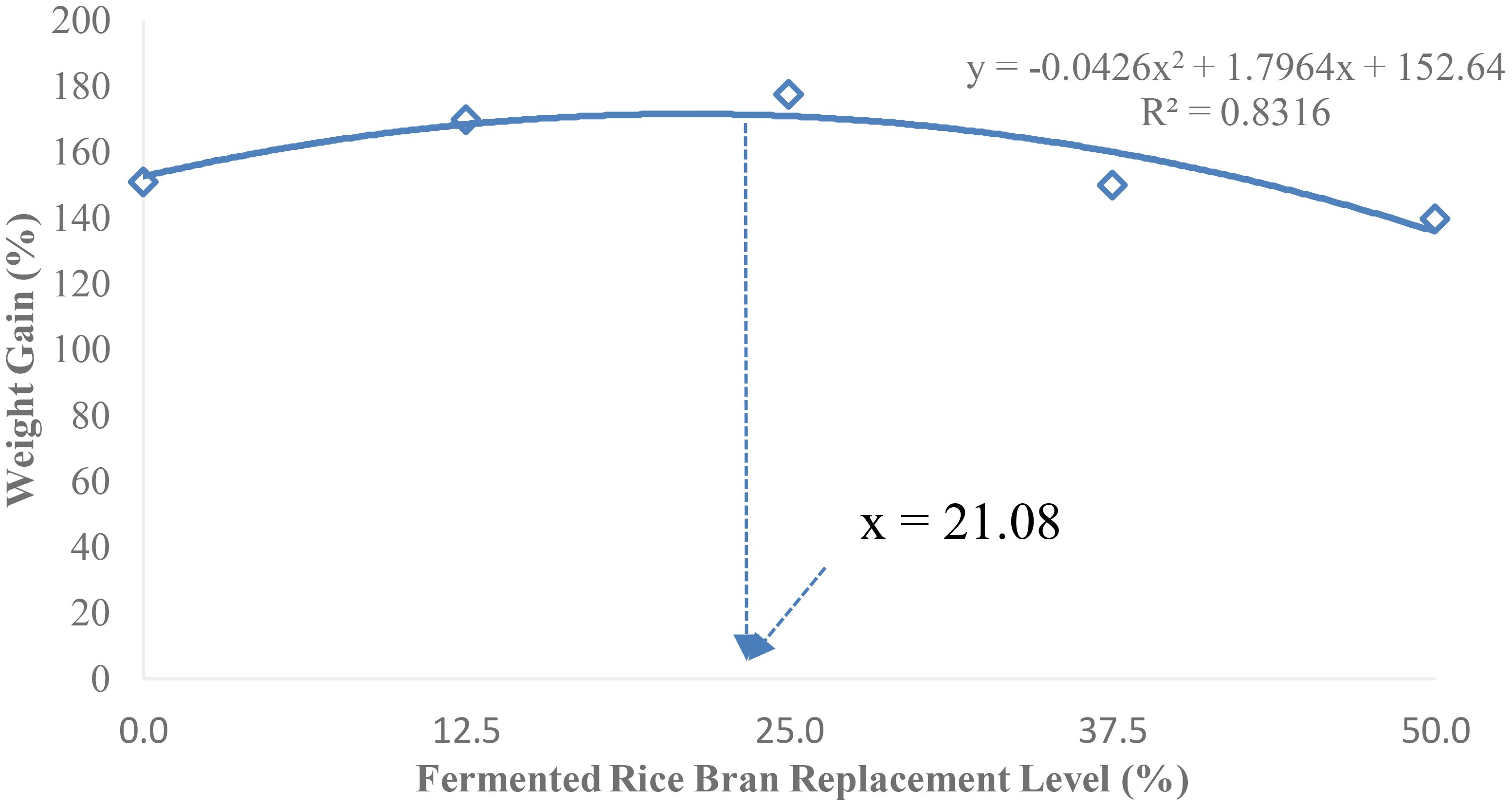
Figure 1 Optimum soybean meal replacement level of fermented rice bran (FRB) to achieve maximum growth in P. monodon.
3.5 Proximate and amino acid composition of shrimp carcass
There were no significant differences (P > 0.05) in the whole-body composition of shrimp fed with different levels of FRB (Table 9). The crude protein, crude lipid, ash, and moisture were found to be similar among treatments.
After the feeding trial, it was determined that the T50 diet was comparable to the T0 diet in terms of growth performance and shrimp whole body composition. The amino acid profile of shrimp tail muscle fed with T0 and T50 diets was then analyzed for comparison. The histidine, arginine, and lysine values were reported to be significantly higher (P< 0.05) in P. monodon fed with a T50 diet compared to T0 (Table 10). However, no significant differences (P > 0.05) were observed in the total amino acids, essential amino acids, and non-essential amino acids of P. monodon when fed with T50 compared to T0.
3.6 Nutrient retention
Protein retention in shrimp fed with increasing FRB replacement levels was found to be significantly different (P< 0.05) among treatments (Figure 2). Shrimp fed with the T25 diet exhibited the highest protein retention (12.46 ± 0.05), followed by those fed with the T12.5 diet (10.35 ± 0.11), both of which were significantly higher than those fed with the T0 diet (9.35 ± 0.08), T37.5 diet (9.32 ± 0.3), and T50 diet (8.97 ± 0.04). The protein retention of shrimp fed with the T37.5 diet and T50 diet was not significantly different than the T0 diet. In contrast, FRB dietary protein replacement did not significantly (P > 0.05) influence lipid retention of P. monodon juveniles.
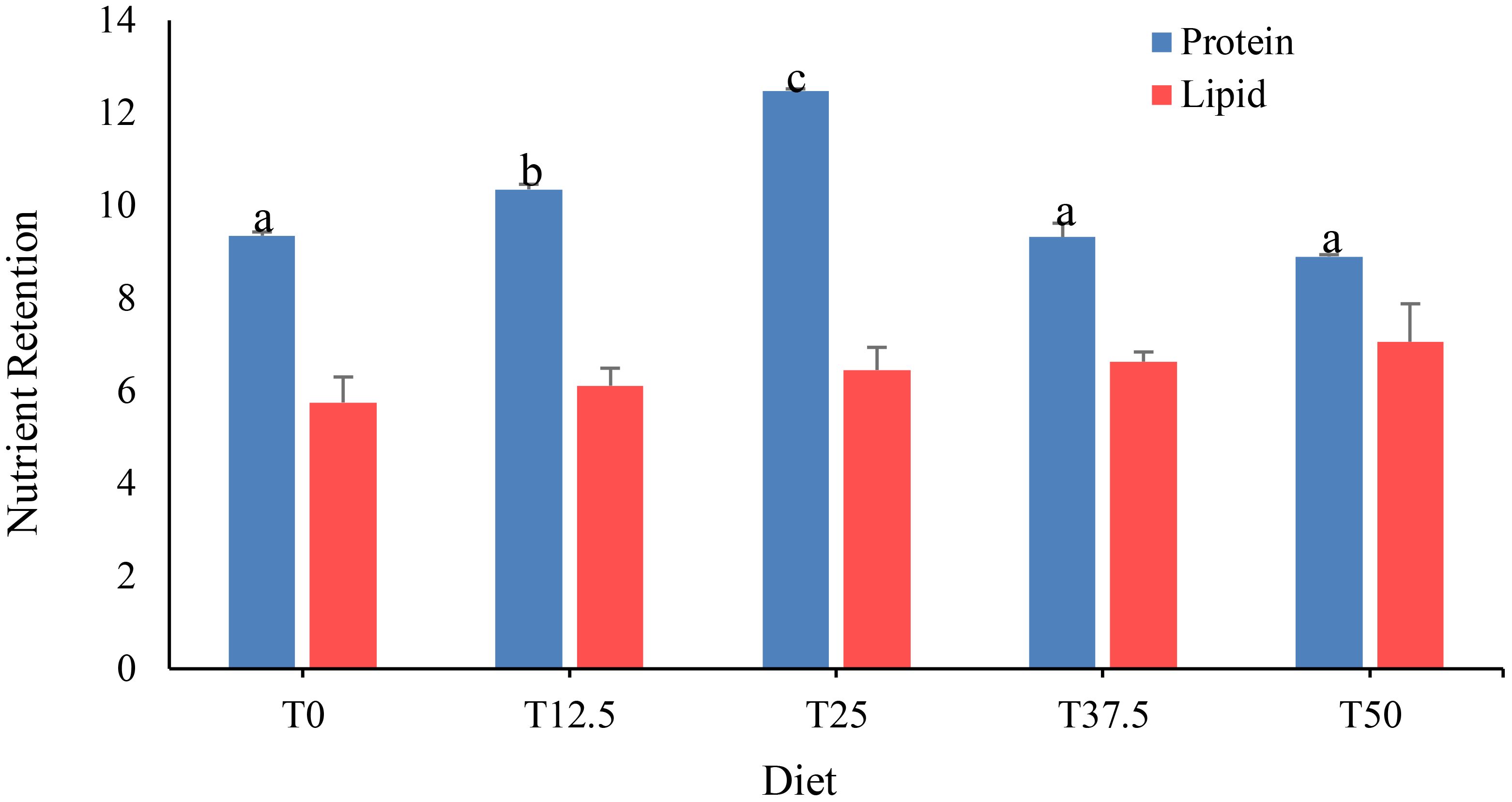
Figure 2 Nutrient retention of Peneus monodon fed with different levels of fermented rice bran (FRB).
3.7 Water quality parameters
The mean water temperature was 24.84 ± 0.03°C (range: 24.8-25.8°C) at 0800H while 27.14 ± 0.18°C (range: 25.8-27.8°C) at 1600H. The lowest recorded water temperature was at 24.8°C, while the highest was at 27.8°C. The mean dissolved oxygen (DO) was 4.84 ± 0.07 mg/l (range 4.75-6.25 mg/l), the mean salinity was 15.14 ± 0.06 ppt (range: 14-16 ppt), and the mean pH was 8.20 ± 0.01 (range: 8.12-8.30). The average ammonia was 0.47 ± 0.12 ppm (range: 0.25-1.00 ppm), average nitrite was 0.57 ± 0.44 ppm (range: 0-2 ppm), and average nitrate was 1.5 ± 0.33 ppm (range: 0-10 ppm).
4 Discussion
The nutritional composition of rice bran was improved after fermentation by T. harzianum. The protein content was increased to about 34.5%, and the fiber was decreased to about 2.1%. Rice bran fermentation has been shown to improve its nutritional composition. The application of solid-state fermentation (SSF) by T. harzianum using rice polishing as a substrate was demonstrated in the study of Ahmed et al. (2017), where higher proteins were also attained. Solid-state fermentation appears to improve the nutritional value by improving the digestibility and increasing the protein content of the biomass material. Nutrient enrichment of rice bran was also reported in other studies using different types of fungus, Trichoderma viride (Iyayi and Aderolu, 2004), Rhizopus oryzae (Oliveira et al., 2010; Schmidt and Furlong, 2012), Pleurotus sapidus (Omarini et al., 2019), Trichoderma longibrachiatum and Aspergillus niger (Hong et al., 2016). Improvement of nutritional composition was also revealed in other agro-industrial waste like fermented sweet potato meal (Traifalgar et al., 2019), fermented copra meal (Apines-Amar et al., 2016), and fermented palm kernel cake (Yana et al., 2010). The improvement in protein content due to fermentation has been associated with the bioconversion of starchy substrates into protein-rich microbial cellular components (Jaganmohan et al., 2013).
In the present study, fermentation decreased the fiber content of rice bran by about 7-fold compared to the unfermented rice bran. The content of total dietary fiber (TDF) in rice bran is approximately 20-30%, and nearly 90% of that content consists of insoluble dietary fiber (IDF) comprising of cellulose, hemicellulose, insoluble β-glucan, and arabinoxylans (Lai et al., 2007; Zhao et al., 2018). The high content of these IDF in rice bran is responsible for the low nutritional value and limited use of this biomass in feeds (Dodd and Cann, 2009). Fermentation using fungi has been known to decrease the IDF of agricultural biomass since these organisms are known producers of xylanase enzymes that are more active than those produced by yeasts and bacteria (Ravindra, 2001; Polizeli et al., 2005). Among these fungi, T. harzianum has been reported to produce cellulases and hemicellulases that are active in hydrolyzing plant cellulose. This may explain the decreased fiber content of rice bran due to fermentation in the present study (Kim et al., 2003; Gottschalk et al., 2010; Pathak et al., 2014).
The nutritional quality of a feed ingredient for farmed animals is quantified based on the digestibility coefficient that measures the availability of nutrients for assimilation. Results suggest that the SSF of rice bran has improved the digestibility coefficient of this ingredient. The apparent ingredient digestibility coefficient for dry matter and protein of P. monodon was about 85% and 90%, respectively. These values are higher than those of raw, unprocessed rice bran and soybean meal in L. vannamei (Akiyama, 1989; Akiyama et al., 1989). This improvement in digestibility could be attributed to the decrease in cellulosic polysaccharides that intervene in the enzymatic digestion of food nutrients (Singh et al., 2009). Enzymes secreted by T. harzianum may also explain the high protein digestibility index of the fermented rice bran in the present study (Polizeli et al., 2005).
The quality of protein in a fish diet depends on the amount and balance of amino acids, which impact growth and production costs. Furthermore, besides its crucial role in protein synthesis and nitrogen balance, it also plays a significant part in vital metabolic processes in fish (Jobgen et al., 2006). Results of the present study showed that SSF with T. harzianum increased the protein content of rice bran about 3-fold compared to the raw material. The quantity of total amino acids in FRB was also increased as compared to the unfermented rice bran, indicating an improvement in the quality of protein. Similar improvements in the protein content and amino acid profile were observed when rice bran was fermented using A. niger (Putra et al., 2022). The beneficial effect of SSF was also reported in fermented copra meal (Dairo and Fasuyi, 2008), fermented sweet potato meal (Traifalgar et al., 2019), and fermented rice bran (Joseph et al., 2008). This improvement in protein content and quality has been associated with the microbial biomass that is known as natural protein concentrate as it contains highly digestible proteins with complete essential amino acids (Kurbanoğlu, 2001; Aggelopoulos et al., 2014).
The essential amino acid index of FRB was found to be high at 84%, rated as a good quality protein material, and comparable to soybean meal (Oser, 1959; Peñaflorida, 1989). The chemical score index of FRB showed tryptophan as the limiting amino acid. The amino acid profile of the fermented material is dictated by the microbial species and substrate used in the fermentation (Denardi-Souza et al., 2018). Trichoderma utilizes tryptophan to form indole acetic acid, and the utilization of this amino acid by this fungus may explain the low tryptophan level in fermented rice bran (Kumar et al., 2017). In addition, deficiency in tryptophan among plant protein sources also agreed with the findings of the previous studies (Fetuga et al., 1973; Felker and Bandurski, 1977).
The use of soybean meal as a major plant protein source is considered a standard in aquatic animal nutrition. The results of the present study confirm the viability of FRB in replacing SBM in the diet of juvenile P. monodon. FRB substitution of 25% soybean meal in the diet of P. monodon showed significant improvement in weight gain, specific growth rate, FCR, and PER. However, no significant effect on growth performance was observed when replacing soybean meal at higher levels. This indicates that fermentation could improve the nutritional value of rice bran and be used as a partial replacement for soybean meal in the diet of P. monodon. The results of this study also showed higher replacement levels of FRB when compared to another study where only 20% of soybean meal was replaced by FRB utilizing A. niger in catfish diets (Putra et al., 2022). Other studies have also reported the positive effects of replacing soybean meal with fermented agro-industrial wastes on shrimp growth. For example, L. vannamei exhibited improved growth when fed diets containing fermented sweet potato meal (Traifalgar et al., 2019), while fermented copra meal was identified as a practical alternative protein source for black tiger shrimp diets (Apines-Amar et al., 2016). Moreover, our results align with previous studies on terrestrial animals, which have demonstrated enhancements in growth performance across various species; growth of broiler chickens fed with Saccharomyces cerevisiae FRB (Kang et al., 2015), higher egg production in layers-fed diet with T. viride FRB (Iyayi and Aderolu, 2004) and reduced feed cost in pigs fed with T. longibrachiatum, A. niger, Pichia kudriavzevii and Lactobacillus buchneri FRB (Hong et al., 2016).
Results on the carcass composition indicate no negative influence of FRB in the nutritional composition of P. monodon. Furthermore, the protein retention in shrimp was improved when SBM was replaced up to 25%. However, higher SBM replacement showed retention levels similar to the control. This could be explained by the increased essential amino acids in the diets with FRB, which led to efficient protein retention. This result is in contrast to other studies where partial replacement of SBM by fermented agro-industrial wastes showed no significant effect on the protein retention of shrimp (Apines-Amar et al., 2016; Traifalgar et al., 2019).
The amino acid analysis of P. monodon juveniles after the feeding trial showed that lysine in shrimp fed with 50% FRB replacement of SBM was significantly higher than control. Lysine, along with proline, alanine, glycine, serine, glutamic acid, and leucine, have been shown to be the important taste compounds of shrimp (Raksakulthai and Norman, 1992). The increase in these amino acids would further enhance its desirable flavor, and the decline can cause changes in the sensory characteristics of shrimp (Peralta et al., 2008). Furthermore, the glutamic acid, a substance responsible for the ‘umami’ taste in fish products (Lopetcharat et al., 2001; Kim et al., 2003) of 50% FRB-fed P. monodon, was higher than the control. These results suggest that FRB could improve the sensory characteristics of P. monodon, as shown by an increase in the amount of amino acid important to shrimp taste.
The present study has demonstrated that solid-state fermentation could improve the nutritional value of rice bran. Fermentation has increased the protein, decreased the fiber contents, enhanced the amino acid profile, and improved the digestibility coefficient of this feed ingredient. Nutritional evaluation tests through a feeding trial indicate that fermented rice bran could partially replace dietary soybean meal without affecting the growth performance and biochemical composition of P. monodon. Furthermore, a 25% replacement of SMB by FRB could improve the growth of P. monodon. It is recommended that 50% replacement of FRB could replace soybean meal without affecting the growth of shrimp. However, further research is required to explore the complete substitution of soybean meal with fermented rice bran. Given that rice bran is abundantly produced as a by-product of the rice industry in Asian countries, utilizing fermented rice bran as a feed ingredient represents a renewable and eco-friendly approach to achieving the sustainable production of P. monodon in the Asian region.
Data availability statement
The raw data supporting the conclusions of this article will be made available by the authors, without undue reservation.
Ethics statement
Ethical approval was not required for the study involving animals in accordance with the local legislation and institutional requirements because this study adheres to the Philippine National Standard (PNS) on the Code of Good Aquaculture Practices (GAqP) for Shrimp and Crab (BAFS, 2017). Protocols on rearing, handling, and animal welfare, were strictly followed based on the guidelines stipulated in Philippine Republic Act Number 8485, known as the Animal Welfare Act of 1998.
Author contributions
FH: Conceptualization, Data curation, Formal analysis, Funding acquisition, Investigation, Methodology, Project administration, Resources, Software, Supervision, Validation, Visualization, Writing – original draft, Writing – review & editing. RT: Conceptualization, Data curation, Funding acquisition, Investigation, Methodology, Resources, Supervision, Validation, Visualization, Writing – review & editing. CD: Data curation, Writing – review & editing, Software.
Funding
The author(s) declare financial support was received for the research, authorship, and/or publication of this article. This research were funded by the Department of Science and Technology - Science Education Institute (DOST-SEI) and the Department of Science and Technology - Philippine Council for Agriculture, Aquatic and Natural Resources Research and Development (DOST-PCAARRD).
Acknowledgments
Acknowledgment is extended to DOST-SEI and DOST-PCAARRD (Mycofeeds for Aquaculture Project), as well as to the Institute of Aquaculture, College of Fisheries and Ocean Sciences, and the Office of the Vice Chancellor for Research and Extension of the University of the Philippines Visayas, for their invaluable support essential to the success of this study.
Conflict of interest
The authors declare that the research was conducted in the absence of any commercial or financial relationships that could be construed as a potential conflict of interest.
Publisher’s note
All claims expressed in this article are solely those of the authors and do not necessarily represent those of their affiliated organizations, or those of the publisher, the editors and the reviewers. Any product that may be evaluated in this article, or claim that may be made by its manufacturer, is not guaranteed or endorsed by the publisher.
References
Aggelopoulos T., Katsieris K., Bekatorou A., Pandey A., Banat I. M., Koutinas A. A. (2014). Solid state fermentation of food waste mixtures for single cell protein, aroma volatiles and fat production. Food Chem. 145, 710–716. doi: 10.1016/j.foodchem.2013.07.105
Ahmed S., Bashir A., Saleem H., Saadia M., Jamil A. (2009). Production and purification of cellulose-degrading enzymes from a filamentous fungus Trichoderma harzianum. Pak J. Bot. 41, 1411–1419.
Ahmed S., Mustafa G., Arshad M., Rajoka M. I. (2017). Fungal biomass protein production from Trichoderma harzianum using rice polishing. BioMed. Res. Int. 2017, e6232793. doi: 10.1155/2017/6232793
Akiyama D. M. (1989). Soybean meal utilization by marine shrimp., in AOCS World Congress on Vegetable Protein Utilization in Human Food and Animal Feedstuffs. (Singapore: American Soybean Association), 27.
Akiyama D. M., Coelho S. R., Lawrence A. L., Robinson E. H. (1989). Apparent digestibility of feedstuffs by the marine shrimp Penaeus vannamei Boone. Nippon Suisan Gakkaishi= Bull. Japanese Soc. Sci. Fisheries 55, 91–98. doi: 10.2331/suisan.55.91
Akiyama D. M., Dominy W. G., Lawrence A. L. (1992). “Chapter 25 - Penaeid Shrimp Nutrition,” in Marine Shrimp Culture, eds. Fast A. W., Lester L. J. (Amsterdam: Elsevier), 535–568. doi: 10.1016/B978-0-444-88606-4.50031-X
Aksnes A., Opstvedt J. (1998). Content of digestible energy in fish feed ingredients determined by the ingredient-substitution method. Aquaculture 161, 45–53. doi: 10.1016/S0044-8486(97)00255-X
AOAC (1984). Official Methods of Analysis of the Association of Official Analytical Chemists,. 14th Edn (Arlington: Virginia, USA: Association of Official Analytical Chemists).
AOAC (1990). Official Methods of Analysis of the Association of Official Analytical Chemists, 15th ed. 5th Edn (Arlington: Virginia, USA: Association of Official Analytical Chemists).
Apines-Amar M. J. S., Andrino-Felarca K. G. S., Cadiz R. E., Corre V. L. Jr., Calpe A. T. (2016). Effects of partial replacement of fish meal by fermented copra meal on the growth and feed efficiency in black tiger shrimp, Penaeus monodon. The Israeli Journal of Aquaculture-Bamidgeh 68, 1244–1249. doi: 10.46989/001c.20837
BAFS. (2017). Philippine national standard: Code of Good Aquaculture Practices (GAqP) for Shrimp and Crab. Available at: https://bafs.da.gov.ph/bafs_admin/admin_page/pns_file/PNS%20BAFS%20197_2017%20-%20Code%20of%20Good%20Aquaculture%20Practices%20for%20Shrimp%20and%20Crab.pdf (Accessed November 14, 2022).
Bautista-Teruel M. N., Eusebio P. S., Welsh T. P. (2003). Utilization of feed pea, Pisum sativum, meal as a protein source in practical diets for juvenile tiger shrimp, Penaeus monodon. Aquaculture 225, 121–131. doi: 10.1016/S0044-8486(03)00284-9
Brown P. B., Kaushik S. J., Peres H. (2008). “Protein feedstuffs originating from soybeans,” in Alternative Protein Sources in Aquaculture Diets (New York, USA: The Haworth Press, Inc.), 205–223.
Bulgari D., Alias C., Peron G., Ribaudo G., Gianoncelli A., Savino S., et al. (2023). Solid-state fermentation of Trichoderma spp.: A new way to valorize the agricultural digestate and produce value-added bioproducts. J. Agric. Food Chem. 71, 3994–4004. doi: 10.1021/acs.jafc.2c07388
Bureau D. P., Hua K. (2006). Letter to the editor of aquaculture. Aquaculture 2, 103–105. doi: 10.1016/j.aquaculture.2006.01.028
Castell J. D., Tiews K. (1980). Report of the EIFAC, IUNS and ICES Working Group on Standardization of Methodology in Fish Nutrition Research, Hamburg, Federal Republic of Germany (Rome, Italy: FAO).
Cho C., Slinger S. (1979). Apparent digestibility measurement in feedstuffs for rainbow trout., in Halver J. O., Tiews K., Eds., World Symposium on Fin Fish Nutrition and Fish Feed Technology, (Heenemann Verlagsgesellschaft), 239–247.
Cho C. Y., Slinger S. J., Bayley H. S. (1982). Bioenergetics of salmonid fishes: energy intake, expenditure and productivity. Comparative Biochemistry and Physiology Part B: Comparative Biochemistry 73, 25–41. doi: 10.1016/0305-0491(82)90198-5
Dairo F., Fasuyi A. (2008). Evaluation of fermented palm kernel meal and fermented copra meal proteins as substitute for soybean meal protein in laying hens diets. J. Cent. Eur. Agric. 9, 35–44.
da Silveirai C. M., Furlong E. B. (2007). Characterization of nitrogenated compounds in solid state fermented bran. Ciec. e Tecnologia Alimentos 27, 805–811. doi: 10.1590/S0101-20612007000400021
Denardi-Souza T., Massarolo K. C., Tralamazza S. M., Badiale-Furlong E. (2018). Monitoring of fungal biomass changed by Rhizopus oryzae in relation to amino acid and essential fatty acids profile in soybean meal, wheat and rice. CyTA - J. Food 16, 156–164. doi: 10.1080/19476337.2017.1359676
Deshimaru O., Shigeno K. (1972). Introduction to the artificial diet for prawn Penaeus japonicus. Aquaculture 1, 115–133. doi: 10.1016/0044-8486(72)90013-0
Divakaran S., Obaldo L. G., Forster I. P. (2002). Note on the methods for determination of chromic oxide in shrimp feeds. J. Agric. Food Chem. 50, 464–467. doi: 10.1021/jf011112s
Dodd D., Cann I. K. (2009). Enzymatic deconstruction of xylan for biofuel production. Gcb Bioenergy 1, 2–17. doi: 10.1111/j.1757-1707.2009.01004.x
FAO (2023). World Food and Agriculture – Statistical Yearbook 2023. (Rome, Italy: FAO). doi: 10.4060/cc8166en
FAOSTAT (2024) FAO Fisheries & Aquaculture - Global aquaculture production Quantity, (1950 - 2021). Available at: https://www.fao.org/fishery/statistics-query/en/aquaculture/aquaculture_quantity (Accessed February 6, 2024).
Felker P., Bandurski R. S. (1977). Protein and amino acid composition of tree legume seeds. J. Sci. Food Agric. 28, 791–797. doi: 10.1002/jsfa.2740280904
Fetuga B. L., Babatunde G. M., Oyenuga V. A. (1973). Protein quality of some Nigerian feedstuffs. I. Chemical assay of nutrients and amino acid composition. J. Sci. Food Agric. 24, 1505–1514. doi: 10.1002/jsfa.2740241206
Furukawa A. (1966). On the acid digestion method for the determination of chromic oxide as an index substance in the study of digestibility of fish feed. Nippon Suisan Gakkaishi 32, 502–506. doi: 10.2331/suisan.32.502
Gottschalk L. M. F., Oliveira R. A., da Silva Bon E. P. (2010). Cellulases, xylanases, β-glucosidase and ferulic acid esterase produced by Trichoderma and Aspergillus act synergistically in the hydrolysis of sugarcane bagasse. Biochem. Eng. J. 51, 72–78. doi: 10.1016/j.bej.2010.05.003
Hong T. T. T., An L. V., Be P. T., Lindberg J. E. (2016). Effect of fermented rice bran and cassava waste on growth performance and meat quality of crossbred pigs. World J. Agric. Res. 4, 132–138. doi: 10.12691/wjar-4-5-1
Hung L. T., Quy O. M. (2013). “On-farm feeding and feed management in whiteleg shrimp (Litopenaeus vannamei) farming in Viet Nam, in Hasan M. R., New M. B., eds. On-farm feeding and feed management in aquaculture(Rome, Italy: FAO Fisheries and Aquaculture Technical Paper No. 583.), 357.
Iyayi E. A., Aderolu Z. A. (2004). Enhancement of the feeding value of some agro-industrial by-products for laying hens after their solid state fermentation with Trichoderma viride. Afr. J. Biotechnol. 3, 182–185. doi: 10.5897/AJB2004.000-2032
Jaganmohan P., Daas B. P., Prasad S. V. (2013). Production of single cell protein (SCP) with Aspergillus terreus using solid state fermentation. Eur. J. Biol. Sci. 5, 38–43.
Jang H.-D., Yang S.-S. (2008). Polyunsaturated fatty acids production with a solid-state column reactor. Bioresource Technol. 99, 6181–6189. doi: 10.1016/j.biortech.2007.12.024
Jobgen W. S., Fried S. K., Fu W. J., Meininger C. J., Wu G. (2006). Regulatory role for the arginine–nitric oxide pathway in metabolism of energy substrates. J. Nutr. Biochem. 17, 571–588. doi: 10.1016/j.jnutbio.2005.12.001
Joseph I., Paul R., Bhatnagar D. (2008). Effect of solid state fermentation on nutrient composition of selected feed ingredients. Indian J. Fisheries 55, 327–332.
Kang H. K., Kim J. H., Kim C. H. (2015). Effect of dietary supplementation with fermented rice bran on the growth performance, blood parameters and intestinal microflora of broiler chickens. Eur. Poultry Sci. 79, 1–11. doi: 10.1399/eps.2015.112
Kim K.-C., Yoo S.-S., Oh Y.-A., Kim S.-J. (2003). Isolation and characteristics of Trichoderma harzianum FJI producing cellulases and xylanase. J. Microbiol. Biotechnol. 13, 1–8.
Kumar N. V., Rajam K. S., Rani M. E. (2017). Plant growth promotion efficacy of indole acetic acid (IAA) produced by a mangrove associated fungi-trichoderma viride VKF3. Int. J. Curr. Microbiol. Appl. Sci. 6, 2692–2701. doi: 10.20546/ijcmas
Kurbanoğlu E. B. (2001). Production of single-cell protein from ram horn hydrolysate. Turk J. Biol. 25, 371–377.
Lai V. M., Lu S., He W. H., Chen H. H. (2007). Non-starch polysaccharide compositions of rice grains with respect to rice variety and degree of milling. Food Chem. 101, 1205–1210. doi: 10.1016/j.foodchem.2006.03.024
Llames C. R., Fontaine J. (1994). Determination of amino acids in feeds: collaborative study. J. AOAC Int. 77, 1362–1402. doi: 10.1093/jaoac/77.6.1362
Lopetcharat K., Choi Y. J., Park J. W., Daeschel M. A. (2001). Fish sauce products and manufacturing: A review. Food Rev. Int. 17, 65–88. doi: 10.1081/FRI-100000515
Luh B., Mickus R. R. (1991). Rice Utilization Vol II. (Boston, USA: Springer). doi: 10.1007/978-1-4899-3754-4
Millamena O. M., Bautista M. N., Reyes O. S., Kanazawa A. (1997). Threonine requirement of juvenile marine shrimp Penaeus monodon. Aquaculture 151, 9–14. doi: 10.1016/S0044-8486(96)01486-X
Millamena O. M., Bautista-Teruel M. N., Kanazawa A. (1996a). Methionine requirement of juvenile tiger shrimp Penaeus monodon Fabricius. Aquaculture 143, 403–410. doi: 10.1016/0044-8486(96)01270-7
Millamena O. M., Bautista-Teruel M. N., Kanazawa A. (1996b). Valine requirement of postlarval tiger shrimp, Penaeus monodon Fabricius. Aquaculture Nutr. 2, 129–132. doi: 10.1111/j.1365-2095.1996.tb00051.x
Millamena O. M., Bautista-Teruel M. N., Reyes O. S., Kanazawa A. (1998). Requirements of juvenile marine shrimp, Penaeus monodon (Fabricius) for lysine and arginine. Aquaculture 164, 95–104. doi: 10.1016/S0044-8486(98)00179-3
Millamena O. M., Teruel M. B., Kanazawa A., Teshima S. (1999). Quantitative dietary requirements of postlarval tiger shrimp, Penaeus monodon, for histidine, isoleucine, leucine, phenylalanine and tryptophan. Aquaculture 179, 169–179. doi: 10.1016/S0044-8486(99)00160-X
Oliveira M., dos S., Feddern V., Kupski L., Cipolatti E. P., Badiale-Furlong E., et al. (2010). Physico-chemical characterization of fermented rice bran biomass Caracterización fisico-química de la biomasa del salvado de arroz fermentado. CyTA–Journal Food 8, 229–236. doi: 10.1080/19476330903450274
Omarini A. B., Labuckas D., Zunino M. P., Pizzolitto R., Fernández-Lahore M., Barrionuevo D., et al. (2019). Upgrading the nutritional value of rice bran by Solid-State fermentation with Pleurotus sapidus. Fermentation 5, 44. doi: 10.3390/fermentation5020044
Oser B. L. (1959). ““An integrated essential amino acid index for predicting the biological value of proteins,”,” in Protein and amino acid nutrition (Academic Press Inc, 111 Fifth Avenue, New York). doi: 10.1016/B978-0-12-395683-5.50014-6
Pathak P., Bhardwaj N. K., Singh A. K. (2014). Production of crude cellulase and xylanase from Trichoderma harzianum PPDDN10 NFCCI-2925 and its application in photocopier waste paper recycling. Appl. Biochem. Biotechnol. 172, 3776–3797. doi: 10.1007/s12010-014-0758-9
Peñaflorida V. D. (1989). An evaluation of indigenous protein sources as potential component in the diet formulation for tiger prawn, Penaeus monodon, using essential amino acid index (EAAI). Aquaculture 83, 319–330. doi: 10.1016/0044-8486(89)90043-4
Peralta E. M., Hatate H., Kawabe D., Kuwahara R., Wakamatsu S., Yuki T., et al. (2008). Improving antioxidant activity and nutritional components of Philippine salt-fermented shrimp paste through prolonged fermentation. Food Chem. 111, 72–77. doi: 10.1016/j.foodchem.2008.03.042
Phongthai S., Homthawornchoo W., Rawdkuen S. (2017). Preparation, properties and application of rice bran protein: A review. Int. Food Res. J. 24, 25–34.
Polizeli M., Rizzatti A. C. S., Monti R., Terenzi H. F., Jorge J. A., Amorim D. S. (2005). Xylanases from fungi: properties and industrial applications. Appl. Microbiol. Biotechnol. 67, 577–591. doi: 10.1007/s00253-005-1904-7
PSA (2024). Available at: https://openstat.psa.gov.ph/PXWeb/pxweb/en/DB/DB:2E:FS/0092E4GVAP1.px/table/tableViewLayout1/?rxid=bdf9d8da-96f1-4100-ae09-18cb3eaeb313 (Accessed February 6, 2024). PX-Web - Table.
Putra A. N., Syamsunarno M. B., Rohayati D., Sarah Y. M., Matien G. A. (2022). Evaluation of fermented rice bran to replace soybean meal in the diet of Clarias sp. fingerling. Jurnal Akuakultur Indonesia 21, 142–151. doi: 10.19027/jai.21.2.142-151
Raksakulthai N., Norman H. F. (1992). Correlation between the concentration of peptides and amino acids and the flavour of fish sauce. ASEAN Food J. 7, 86–90.
Ravindra P. (2001). Studies on production of single cell protein by Aspergillus niger in solid state fermentation of rice bran. Braz. Arch. Biol. Technol. 44, 79–88. doi: 10.1590/S1516-89132001000100011
Sala A., Barrena R., Artola A., Sánchez A. (2019). Current developments in the production of fungal biological control agents by solid-state fermentation using organic solid waste. Crit. Rev. Environ. Sci. Technol. 49, 655–694. doi: 10.1080/10643389.2018.1557497
Schmidt C. G., Furlong E. B. (2012). Effect of particle size and ammonium sulfate concentration on rice bran fermentation with the fungus Rhizopus oryzae. Bioresource Technol. 123, 36–41. doi: 10.1016/j.biortech.2012.07.081
Singh S., Tyagi C. H., Dutt D., Upadhyaya J. S. (2009). Production of high level of cellulase-poor xylanases by wild strains of white-rot fungus Coprinellus disseminatus in solid-state fermentation. N Biotechnol. 26, 165–170. doi: 10.1016/j.nbt.2009.09.004
Supriyati T. H., Susanti T., Susana I. W. R. (2015). Nutritional value of rice bran fermented by Bacillus amyloliquefaciens and humic substances and its utilization as a feed ingredient for broiler chickens. Asian-Australasian J. Anim. Sci. 28, 231. doi: 10.5713/ajas.14.0039
Tillman A., Reksohadiprodjo S., Prawirokusumo S., dan Lebdosoekojo S. (2005). Basic Animal Nutrition Science. (Yogyakarta, Indonesia: Gadjah Mada University Press).
Traifalgar R. F., Pagapulan J., Valdez M. T., Ellamar J. B., Ocampo E. J. C., Ilag L. L. (2019). Fermented sweet potato meal, a sustainable dietary protein ingredient for juvenile Penaeus vannamei, boone 1931. Israeli J. Aquaculture - Bamidgeh 71, 11. doi: 10.46989/001c.20973
World Agricultural Production (2024) Rice Production by Country | World Agricultural Production 2023/2024. Available at: http://www.worldagriculturalproduction.com/crops/rice.aspx (Accessed February 9, 2024).
Yana S., Umi A., Vitus D. Y., Ejeng S. (2010). Bioconversions of palm kernel cake and rice bran mixtures by Trichoderma viride toward nutritional contents. Int. J. Sci. Eng. 1, 27–32.
Keywords: solid-state fermentation, alternative protein source, Penaeus monodon, rice bran, Trichoderma harzianum, soybean meal
Citation: Huervana FH, Traifalgar RFM and Dionela CS (2024) Solid-state fermentation converts rice bran into a high-protein feed ingredient for Penaeus monodon. Front. Mar. Sci. 11:1384492. doi: 10.3389/fmars.2024.1384492
Received: 09 February 2024; Accepted: 12 April 2024;
Published: 10 May 2024.
Edited by:
Amit Ranjan, Tamil Nadu Fisheries University, IndiaReviewed by:
Vikas Phulia, Guru Angad Dev Veterinary and Animal Sciences University, IndiaSarvendra Kumar, College of Fisheries Kishanganj, India
Copyright © 2024 Huervana, Traifalgar and Dionela. This is an open-access article distributed under the terms of the Creative Commons Attribution License (CC BY). The use, distribution or reproduction in other forums is permitted, provided the original author(s) and the copyright owner(s) are credited and that the original publication in this journal is cited, in accordance with accepted academic practice. No use, distribution or reproduction is permitted which does not comply with these terms.
*Correspondence: Fredson H. Huervana, fhhuervana@up.edu.ph